This page is under revision
Learning Objectives
- Explain the endosymbiotic theory for the origin of eukaryotes
- Use evidence from the endosymbiotic theory to place the origin of mitochondria and chloroplasts on the tree of life
- Identify and describe the key traits and adaptations unique to eukaryotes
- Differentiate between protists and other eukaryotic groups
- Describe and differentiate between the three multicellular eukaryotic life cycles based on the ploidy of the multicellular organism
- Describe the importance of single-celled eukaryotes with respect to human health and ecosystem services
Endosymbiotic Theory for the Origin of Eukaryotes
Microfossil evidence suggests that eukaryotes evolved sometime between 1.6 and 2.2 billion years ago during the Proterozoic. Prior to the origin of eukaryotes, all life on Earth was prokaryotic (lacking nucleus or other membrane-bound organelles). The leading hypothesis for the origin of eukaryotes is called the endosymbiotic theory, where eukaryotes arose as a result of a fusion of archaea cells with bacteria, after an ancient archaea engulfed (but did not eat) an ancient, aerobic bacterial cell. The engulfed (endosymbiosed) bacterial cell remained within the archaea cell in what may have been a mutualistic relationship: the engulfed bacterium allowed the host archean cell to use oxygen to release energy stored in nutrients, and the host cell protected the bacterial cell from predators. Over many generations, a symbiotic relationship developed between the two organisms so completely that neither could survive on its own. The descendants of this ancient, engulfed bacterial cell are present in all eukaryotic cells today asĀ mitochondria.
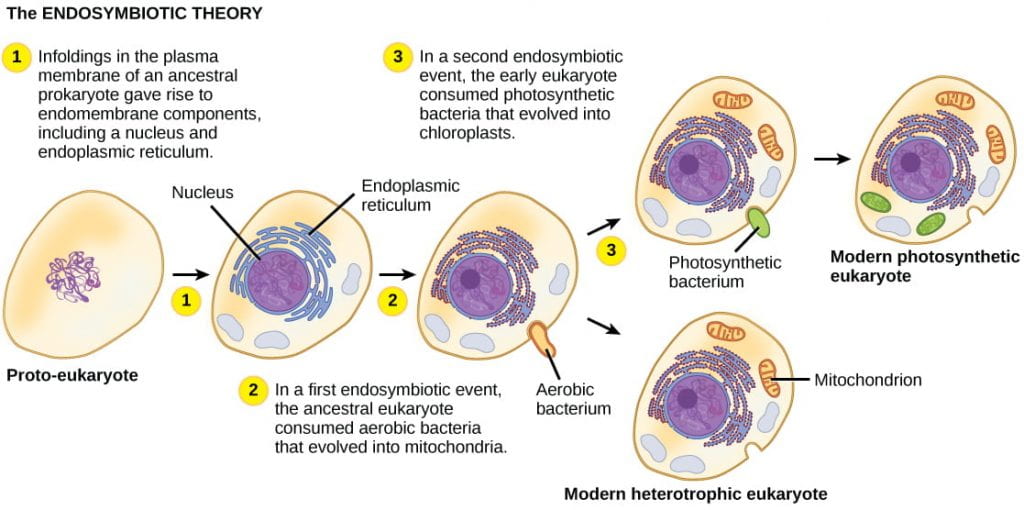
The first eukaryote may have originated from an ancestral prokaryote that had undergone membrane proliferation, compartmentalization of cellular function (into a nucleus, lysosomes, and an endoplasmic reticulum), and the establishment of endosymbiotic relationships with an aerobic prokaryote which led to mitochondria. Some early eukaryotes later engulfed a photosynthetic bacterium similar to cyanobacteria, which led to chloroplasts in modern-day photosynthetic eukaryotes. By Phil Schatz – http://philschatz.com/biology-concepts-book/contents/m45513.html, CC BY-SA 4.0, https://commons.wikimedia.org/w/index.php?curid=68096564
The video below gives an overview of the endosymbiotic theory for the origin of eukaryotes:
The information below was adapted from OpenStax Biology 23.1
A secondary endosymbiosis event occurred in a group of green algae that engulfed a photosynthetic cyanobacterium, leading to the origin of chloroplasts and the evolution of green plants. Evidence suggests that engulfment of a cyanobacteria-like organism has happened at least twice so far in the history of eukaryotes: in one case, the common ancestor of the major lineage/supergroup Archaeplastida took on a cyanobacterial endosymbiont (this is the lineage that includes green plants); in the other, the ancestor of the small amoeboid rhizarian taxon, Paulinella, took on a different cyanobacterial endosymbiont (this group includes freshwater and marine amoebas).
Evidence for the Endosymbiotic Theory for the Origin of Mitochondria and Chloroplasts
There are multiple, independent lines of evidence to support the hypothesis that eukaryotes evolved from an endosymbiotic event between an ancient archaean cell and an ancient aerobic bacterium:
- Mitochondria and chloroplasts are approximately the same size as prokaryotic cells, but they are located inside much larger eukaryotic cells instead of free-living.
- Mitochondria and chloroplasts each have their own DNA, their DNA is organized in a circular chromosome like typical prokaryotic genomes, and their genomes contain genes that are very similar to genes found in prokaryotic genomes.
- Mitochondria and chloroplasts reproduce by binary fission, the process that prokaryotes use to reproduce. In contrast, eukaryotic cells reproduce by mitosis.
- If the mitochondria or chloroplasts are removed from a eukaryotic cell, the cell has no way to produce new ones. In other words, the genetic “instructions” to make new mitochondria/chloroplasts is not present in the eukaryotic nuclear genome; they are present in the mitochondria/chloroplast genomes.
- The membrane composition of mitochondria and chloroplasts is more similar in composition to prokaryotic membranes than to eukaryotic membranes.
How does this evidence map to the tree of life? Since all eukaryotes have mitochondria, but only photosynthetic eukaryotes have chloroplasts, the principle of parsimony (the idea that the explanation requiring the fewest steps is most likely correct) argues that first, an ancestral eukaryote engulfed the bacteria (which led to mitochondria). Secondly, only in the lineage that led to plants and algae, a later descendant of this ancestral eukaryote then engulfed a cyanobacteria-like species that led to chloroplasts. This hypothesis is represented in the phylogenetic tree below:

The origin and evolutionary tree of life that is based on small-subunit RNA. The branches that perform oxygenic photosynthesis are labeled with ‘O2’. The black arrows indicate the endosymbiotic events that resulted in the origin of eukaryotes from proteobacteria-like organisms (ultimately leading to mitochondria), and later eukaryotic photosynthesis from cyanobacteria-like organisms, which ultimately became chloroplasts in algae and later in plants. Image adapted from work by Govindjee, Dmitriy Shevela – doi:10.3389/fpls.2011.00028, CC BY 3.0, https://commons.wikimedia.org/w/index.php?curid=59645694
Unique Traits and Adaptations of Eukaryotes
The information below was adapted from OpenStax Biology 23.1
There are many unique traits and adaptations of eukaryotes that allow us to distinguish them as a monophyletic group on the phylogenetic tree of life. Based on the principle of parsimony, the following lists a subset of traits must have been present in the most recent common ancestor (MRCA) from which all eukaryotic life emerged:
- Cells with nuclei surrounded by a nuclear envelope with nuclear pores: This is the single trait that is both necessary and sufficient to define an organism as a eukaryote. All extant eukaryotes have cells with nuclei.
- Mitochondria: Some extant eukaryotes have very reduced remnants of mitochondria in their cells, whereas other members of their lineages have mitochondria.
- Mitosis: a process of nuclear division wherein replicated chromosomes are divided and separated using elements of the cytoskeleton. Mitosis is universally present in eukaryotes.
- Meiosis and sex: a process of genetic recombination unique to eukaryotes in which diploid nuclei at one stage of the life cycle undergo meiosis to yield haploid nuclei and subsequent karyogamy, a stage where two haploid nuclei fuse together to create a diploid zygote nucleus.
There are eukaryotic species with exceptions to the list above: for example, some species of rotifers (microscopic, aquatic animals) reproduce asexually without meiosis. But in all cases of exceptions, evidence indicates that a particular trait was lost in that lineage rather than the lineage independently evolving all other traits of eukaryotes.
The video below describes the origin and advantages of sexual vs asexual reproduction in eukaryotes:
General Steps of Sexual Life Cycles
The information below was adapted from Wikipedia “Biological life cycle”
Sexual reproduction with meiosis is a defining feature of eukaryotes. Unlike asexual reproduction, where the offspring are genetically identical to their parent, the offspring that result from sexual reproduction get half of their genetic information from two different parents. Sexual reproduction therefore always involves two changes in ploidy (the number of copies of each chromosome):
- The first change in ploidy occurs via meiosis, a cell division that reduces ploidy by 1/2, from “2n” (diploid) to “1n” (haploid), where “n” is the number of copies of each chromosome. This reduction produces haploid cells from diploid cells.
- The second change in ploidy is a doubling from 1n back to 2n (return to original ploidy) by fertilization, or the joining of gametes (typically called egg and sperm).
There are multiple variations on the timing of these steps, and other steps that can happen in between them. We’ll discuss these variations on sexual life cycles much more in depth later in the term, but for now, here is a brief overview of the three sexual life cycles in regard to timing of the changes of ploidy:
- haplontic life cycle: organisms with this life cycle have a multicellular haploid stage (the multicellular organism you see by eye is haploid), and the diploid stage exists only as a single cell (the fertilized egg). This life cycle is typical of most fungi: the large, multicellular mushroom that you can see by eye is usually haploid, not diploid!
- diplontic life cycle: organisms with this life cycle have a multicellular diploid stage (the multicellular organism you see by eye is diploid), and the haploid stage exists only as a single cell (the gametes – typically called sperm or eggs). This life cycle is typical of animals: the multicellular animal that you can see is diploid.
- alternation of generations life cycle (also haplodiplontic life cycle): organisms with this life cycle have both a multicellular diploid stage and a multicellular haploid stage. This life cycle is typical of plants, and there are multiple variations on this life cycle in different plant lineages which we will discuss in future class sessions.

A Protist is any Eukaryote that is not a Plant, Fungus, or Animal
When we think of the term “eukaryote,” we tend to think of large, multicellular things like plants, animals, and fungi. But there are many (many!) eukaryotes that fall outside of these three monophyletic groups; these other eukaryotes are often grouped together as “protists.” A protist is defined as any eukaryote that is not classified as a plant, animal, or fungus. (It is often incorrectly thought to mean any single-celled eukaryote, but this is incorrect; there are many examples of multicellular protists). The term “protists” is evolutionarily problematic because it does not refer to a monophyletic group (much like the term “prokaryote.”)

The Importance of Single-Celled Eukaryotes to Human Health and Environmental Processes
The information below was adapted from OpenStax Biology 23.4
Single-celled eukaryotes function in various ecological niches. Whereas some single-celled eukaryotes are essential components of the food chain and generators of biomass, others function in the decomposition of organic materials. Still others are dangerous human pathogens or causative agents of devastating plant diseases. Below we highlight a few examples of single-celled eukaryotes that impact humans positively or negatively:
- Human pathogens and parasites: A pathogen is anything that causes disease. Parasites live in or on an organism and harm the organism.
- Plasmodium Species: Members of the genus Plasmodium cause malaria; they must colonize both a mosquito and a vertebrate to complete their life cycle. In vertebrates, the parasite develops in liver cells and goes on to infect red blood cells, bursting from and destroying the blood cells with each asexual replication cycle. Of the four Plasmodium species known to infect humans, P. falciparum accounts for 50 percent of all malaria cases and is the primary cause of disease-related fatalities in tropical regions of the world.
- Trypanosomes: Trypanosoma brucei, the parasite that is responsible for African sleeping sickness, escapes immune detection by changing its thick layer of surface glycoproteins with each infectious cycle. The glycoproteins are identified by the immune system as foreign antigens, and a specific antibody defense is mounted against the parasite. However, T. brucei has thousands of possible antigens, and with each subsequent generation, the protist switches to a glycoprotein coating with a different molecular structure. In this way, T. brucei is capable of replicating continuously without the immune system ever succeeding in clearing the parasite. Without treatment, T. brucei attacks red blood cells, causing the patient to lapse into a coma and eventually die.
- Plant pathogens and parasites: Plant pathogens and parasites negatively impact plant health in ways that can immediately impact humans through destruction of food crops:
- Phytophthora infestans is responsible for a disease called potato late blight, which causes potato stalks and stems to decay into black slime. Widespread potato blight caused by P. infestans precipitated the well-known Irish potato famine in the nineteenth century that claimed the lives of approximately 1 million people and led to the emigration of at least 1 million more from Ireland.
- Plasmopara viticola parasitizes grape plants, causing a disease called downy mildew. Grape plants infected with P. viticola appear stunted and have discolored, withered leaves. The spread of downy mildew nearly collapsed the French wine industry in the nineteenth century.
- Photosynthesis: We tend to give plants all the credit for photosynthesis, and this is largely true for photosynthesis on land. But phytoplankton, which are microscopic and single-celled photosynthetic organisms, are the primary producers that form the basis of the food web in aquatic systems. Phytoplankton include eukaryotic photosynthesizers like algae and prokaryotic photosynthesizers like cyanobacteria, but here we will highlight two critical eukaryotic groups:
- Diatoms are single-celled photosynthetic algae that generate about 20 percent of the oxygen produced on the planet each year and contribute nearly half of the organic material found in the oceans.
- Dinoflagellates are a large group of single-celled species. They include photosynthetic algae that are endosymbionts of corals and other marine animals and play an essential role in the biology of coral reefs.
- Decomposition: When organisms die, the nutrients in their bodies are inaccessible to other living things, unless they are biologically ‘broken down’ into simpler organic molecules. This process is carried out by decomposers, without which we would soon run out of many essential nutrients because they would be permanently locked away in dead things (plus we’d run out of space for living things to live!)
- Many types of oomycetes, which are fungus-like protists, grow on dead animals or algae and play an essential role in returning inorganic nutrients to the soil and water. This process allows for new plant growth, which in turn generates sustenance for other organisms along the food chain.