This page is under revision
Learning Objectives
- Place and identify the clade fungi on a phylogenetic tree within the domain Eukarya
- Define traits and adaptations common to fungi
- Describe the general fungal morphology and life cycle of multicellular fungi, and differentiate between single and multicellular fungal morphology
- Describe the metabolic traits of fungi with respect to their ecosystem services and human relevance
- Describe the mutualistic and symbiotic relationship of fungi with land plants, and explain why plant transition to land was facilitated by fungi
Fungi on the Phylogenetic Tree of Life
Fungi are a monophyletic group of eukaryotic heterotrophs that is closely related to animals. As eukaryotes, their cells contain a nucleus, mitochondria, and a complex system of internal membrane including the endoplasmic reticulum and Golgi apparatus. Unlike plant cells, fungal cells do not have chloroplasts or chlorophyll. The lineage that led to fungi and animals is thought to have diverged from the lineage that led to plants approximately 1.5 billion years ago during the Proterozoic Eon, and the lineage that led to fungi is thought to have diverged from the lineage that led to animals approximately 800 to 900 million years ago near the end of the Proterozoic eon. Fungi are more closely related to animals than they are to plants. The common ancestor of all fungi was likely aquatic, flagellated, and single-celled.

Fungi are monophyletic, but the evolutionary relationships among some of the of different groups within fungi are not yet clearly resolved (note the unresolved points of Mucoromycota, Glomeromycota, Chytridiomycota, and Blastocladiomycota in the phylogenetic tree below):

The tree below shows a simplified view of a subset of fungi, again revealing some of the unresolved relationships among these fungal groups (see unresolved branch points below):

Shared Adaptations and Traits Unique to Fungi
The information below was adapted from OpenStax Biology 24.1
Fungi are eukaryotes with an enormous variety of body plans and, along with land plants and animals, are one of the major evolutionary lineages to occupy land. While scientists have identified about 100,000 species of fungi, this is only a fraction of the 1.5 million species of fungus likely present on Earth. Edible mushrooms, yeasts, black mold, and the producer of the antibiotic penicillin, Penicillium notatum, are all fungi. Different species of fungi can be mutualistic, parasitic, or serve as decomposers. Currently the largest (and perhaps, oldest) living organism on Earth’s surface is a fungus: the giant Armillaria solidipes (honey mushroom) is considered the largest organism on Earth, spreading across more than 2,000 acres of underground soil in eastern Oregon; it is estimated to be at least 2,400 years old!
While fungi can be multicellular or unicellular and have a wide range of body plans and lifestyles, all fungi have several traits in common:
- all fungi are heterotrophic eukaryotes, meaning they must use existing organic compounds as a source of carbon
- all fungi possess cell walls made of a tough polysaccharide called chitin*; the cell wall provides structural strength to the fungal body (chitin is also a major component of the exoskeletons of arthropods such as insects, crustaceans, and spiders)
- all fungi engage in external digestion of food, meaning that they excrete digestive enzymes outside of their bodies, and the digested nutrients are then absorbed from the external environment
*In 2011, a new clade of parasitic fungi called Rozellomycota or Cryptomycota (“hidden fungi”) was identified which lacks a cell wall made of chitin. While the lack of chitin seems like it should disqualify this group from being considered fungi, the Cryptomycota do still produce chitin in their spores; it is thought that loss of the chitin cell wall may have been an adaptation of their parasitic lifestyle.
General Fungal Morphology and Life Cycles
The information below was adapted from OpenStax Biology 24.1
In this section we will discuss the typical characteristics of many fungi, but keep in mind, there are often exceptions to the rule.
- Morphology: Most fungi are multicellular; some (like yeast) are unicellular; and some can switch from a unicellular to a multicellular state depending on environmental conditions. Unicellular fungi reproduce by budding, and the new bud separates from the parent cell to form two separate, individual cells. In contrast, multicellular fungi have cells that work together in a body structure called a mycelium (plural: mycelia). The mycelium is composed of branches of cells called hyphae, and it also produces reproductive units (spores) either directly or through a structure called a fruiting body:
- Hyphae: The mycelium is composed of long, branching filamentous (thread-like) structures called hyphae (singular: hypha) that look like roots. The hyphae of most fungi are composed of individual cells that are connected end-to-end in a long, branching thread. Depending on the species, the hyphae composing the mycelium can grow on a surface, in soil or decaying material, in a liquid, or even on living tissue. Hyphae excrete digestive enzymes into the environment and then absorb the nutrients which have been released by digestion.
- Spores/fruiting body: The morphology of the reproductive stage varies dramatically across different groups of fungi; in some groups of fungi, the reproductive stage is highly visible such as a mushroom fruiting body (what we call a mushroom is only the above-ground reproductive structure produced by a massive mycelium composed of hyphae that are almost entirely underground). In other groups of fungi, the reproductive stage is microscopic.

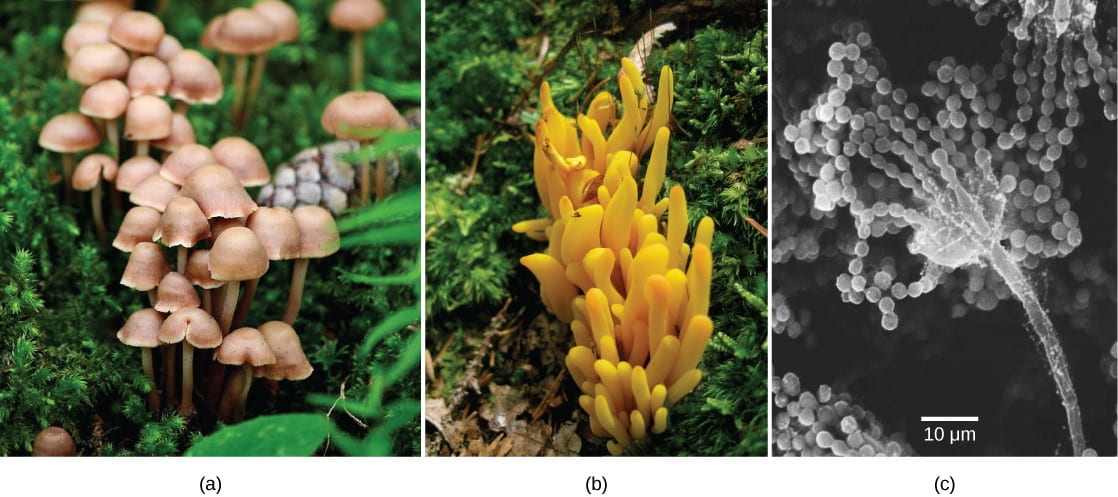
- Life cycle: Some fungi reproduce only asexually, but most fungi are able to reproduce both asexually and sexually via the haplontic life cycle. In the haplontic life cycle, the multicellular stage is haploid, and the diploid stage (the fertilized egg) exists only as a single cell before undergoing meiosis to produce haploid spores. While fungal life cycles can vary widely among different species, the “general” fungal life cycle is described below:
- Vegetative growth (asexual reproduction): The mycelium (the fungal body composed of hyphae), is haploid. The mycelium grows (“germinates”) via mitosis from a haploid spore. The haploid mycelium is capable of continuing to grow via mitosis, or it can reproduce asexually via:
- budding, or controlled growth of a new cell on the side of an existing cell, which then detaches in a controlled manner
- fragmentation, or breaking into smaller pieces, where each piece can then continue growing as an independent organism
- producing its own haploid spores (via mitosis) which can then disperse and grow into new haploid mycelia in new locations. Spores produced mitotically are genetically identical to the parent; in other words, spores produced by mitosis are units of asexual reproduction.
- Sexual reproduction: Sexual reproduction results from the fusing of cells from two mycelia, followed by production of spores by meiosis:
- Plasmogamy and the heterokaryotic stage: To initiate sexual reproduction, haploid cells from two different mycelia fuse together, sharing their cytoplasm (“plasmogamy”); however, their nuclei do not fuse and remain independent in a structure that is “heterokaryotic”, also called a “heterokaryon” or “dikaryon.” Depending on the species, the heterokaryon will grow into a fruiting body (like a mushroom) or will remain microscopic.
- Karyogamy: At this stage, two nuclei within a heterokaryotic cell fuse to form a diploid nucleus. This stage does not grow; it proceeds into the next stage (meiosis) without growth of new cells or tissue.
- Meiosis: The diploid nucleus undergoes meiosis to produce four haploid spores. (Unlike the spores produced by the haploid mycelium, the spores produced from karyogamy are a product of sexual reproduction as the result from the meiotic cell division of a diploid cell.)
- Germination: The meiotically produced spores are dispersed by wind or via animal (depending on the species) to a new environment, where they can germinate into a new mycelium and enter into a new cycle of vegetative growth and/or sexual reproduction.
- Vegetative growth (asexual reproduction): The mycelium (the fungal body composed of hyphae), is haploid. The mycelium grows (“germinates”) via mitosis from a haploid spore. The haploid mycelium is capable of continuing to grow via mitosis, or it can reproduce asexually via:

In both sexual and asexual reproduction as shown above, fungi produce many small, light-weight spores that disperse from the parent organism by either floating on the wind or hitching a ride on an animal. The huge number of spores released increases the likelihood of landing in an environment that will support growth.

Fungal Metabolism: Ecosystem Services and Relevance to Humans
The information below was adapted from OpenStax Biology 24.1
Like animals, fungi are heterotrophic; they use complex organic compounds as a source of carbon, rather than fix carbon dioxide from the atmosphere as do some bacteria and most plants. Below are some common traits among different fungal groups:
- External digestion: Animals ingest food and then digest it in specialized organs; fungi do the reverse: they excrete digestive enzymes out of their hyphae, and these digestive enzymes break down the organic matter in the environment. The hyphae then absorb the nutrients from the digested organic matter; the many hyphae of the mycelium provide an exceptionally large surface area for absorption of nutrients.
- Decomposers: Most fungi are decomposers, which means they derive nutrients from dead or decaying organic matter. Fungal decomposers are also called saprophytic fungi. Fungal exoenzymes are able to break down insoluble polysaccharides, such as the cellulose and lignin of dead wood, into readily absorbable glucose molecules.
- Mutualists: Other fungi form mutualistic relationships with plants, where fungi trade water and key nutrients with plants in exchange for plant sugars. These fungi are called mycorrhizal fungi, and it is estimated that 80-90% of all plant species rely on mycorrhizal fungi for their growth.
- Parasites: Some fungi are parasitic, infecting either plants or animals; for example, smut and Dutch elm disease affect plants, whereas athlete’s foot and candidiasis (thrush) are medically important fungal infections in humans.
- Predators: In environments poor in nitrogen, some fungi even act as predators, trapping small animals like nematodes (a type of roundworm), via a constricting ring within their hyphae that functions like a noose.
In their role as decomposers, saprophytic fungi can break down organic matter which would not otherwise be recycled in the ecosystem. Some elements, such as nitrogen and phosphorus, are required in large quantities by biological systems, yet are not available or abundant in the environment unless this decomposition takes place. Even trace elements present in low amounts in many habitats are essential for growth would remain tied up in dead organic matter if fungi and bacteria did not return them to the environment via their metabolic activity. Thus, fungi make it possible for other living things to be supplied with the nutrients they need to live.
Because of their varied metabolic pathways, humans take advantage of fungi for multiple applications. Not only do they help to stabilize ecosystems and supply us with food, but they are also directly used in the production of beer, cheese, and bread, as well as various medicines such as penicillin. Some fungi are also extremely sensitive to air pollution, especially to abnormal levels of nitrogen and sulfur. The U.S. Forest Service and National Park Service can monitor air quality by measuring their relative abundance and health in an area (read more on this here). Currently, fungi are being investigated as potential tools in bioremediation; for example, some species of fungi can be used to break down diesel oil, polycyclic aromatic hydrocarbons (PAHs), and even heavy metals such as cadmium and lead.
Mutualism and Coevolution with Land Plants
The information below was adapted from OpenStax Biology 24.3
Symbiosis is the ecological interaction between two organisms that live together, and a mutualistic symbiosis is a symbiotic relationship in which both members of the association benefit. Fungi form mutualistic associations with many types of organisms, including cyanobacteria, algae, plants, and animals.
Most terrestrial plants (approximately 80-90%) form mutualistic relationships with fungi via their roots. The roots of the plant connect with the underground parts of the fungus forming mycorrhizae (from the Greek words myco meaning fungus and rhizo meaning root). The relationship is mutually beneficial:
- In a mycorrhizal association, the fungal mycelia use their extensive network of hyphae and large surface area in contact with the soil to increase absorption of both water and minerals from the soil into the plant, including nitrogen and other nutrients that the fungi acquired via decomposition of dead organic matter.
- In exchange, the plant supplies the products of photosynthesis (sugars) to fuel the metabolism of the fungus. Some plants, such as orchids, have developed such a tight biological association with fungi that their seeds generally cannot germinate or grow without a fungal mycorrhiza partner!

Timeline of the Phanerozoic eon, including Eras and Periods, with dates in million years ago (MYA). Image credit: Shana Kerr
The mutualistic relationship between plants and fungi extends to the early evolutionary history of both clades, and the colonization of land by fungi is very much entangled with plants. In fact, plants could not have colonized land ~450 million years ago without the help of fungi:
- In the early Paleozoic Era (the first Era of the Phanerozoic Eon), there was very little soil and most land on Earth was rock; the combined action of early fungi secreting digestive enzymes, coupled with erosion, slowly created soil. Without soil, plants could not have begun growing on land.
- The first land plants where bryophytes (moss-like plants) which lacked true roots and could not survive in permanently dry areas; early fungal associations with these plants helped to provide greater access to water and nutrients in these plants that lacked true roots.
- True roots evolved in seedless vascular plants, and plants with true roots had a selective advantage over bryophytes not only because they could more readily anchor themselves in the soil and extract water, but also because the roots allowed for a greater surface area of contact with fungal partners; this in turn allowed plants with true roots more access to both water and nutrients from the ground and from mutualistic exchange with fungi.
- Over time, the benefits of this interaction led to evolution of present-day mycorrhizae; it is estimated that up to about 90% of today’s vascular plants have associations with fungi in their roots.
For an overview of what you’ve just read, and some new cool tidbits, check out this cool video (Note that the video is a bit outdated, but is otherwise quite on point!):