Learning Objectives
- Describe the formation and structure of soil
- Explain why and how soil composition and texture influences acquisition of water, ions, and minerals by plants
- Define and differentiate between diffusion, facilitated diffusion, ion channels, active transport, proton pumps, and cotransport
- Explain the roles of root hairs, proton pumps, ion channels, co-transporters, and active and passive movement of ions in acquisition of water, ions, and minerals by plants
- Compare and contrast the processes by which Rhizobia bacteria and mycorrhizal fungi facilitate nutrient acquisition by plant roots
Soil Formation, Composition, and Texture
The information below was adapted from OpenStax Biology 31.2
Soil is formed from weathering of rock by mechanical, chemical, and biological processes. Soils differ dramatically in different regions, but all soils consist of both living and nonliving components:
- Humus, or organic matter (living and dead), including plant roots, prokaryotic and eukaryotic microorganisms, decomposing plants/animals; comprises about 5% of soil volume
- Rock fragments and other inorganic mineral matter from rock, slowly broken down into smaller particles that vary in size; comprise about 40 to 45% of soil volume
- Water and gasses (air) which are dissolved in the soil particles; comprises about 50 % of soil volume
The amount of each of the major components of soil depends on the amount of vegetation, soil compaction, and water present in the soil. A good healthy soil has sufficient air, water, minerals, and organic material to promote and sustain plant life.
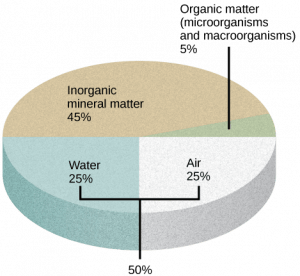
Soil texture is determined by the proportions of differently-sized particles in the soil, which affects both the ability of plant roots to penetrate the soil, and the ability of the soil to hold water. Soils are categorized by texture as follows:
- Gravel: particles larger than 2.0 mm in diameter
- Sand: particles between 0.02 to 2 mm in diameter
- Silt: particles between 0.002 and 0.02 mm
- Clay: particles less than 0.002 mm in diameter
- Loam: soil composed of a a mixture of sand, silt, and humus
Multiple factors influence soil formation, including parent material, climate, topography, biological factors, and time.
- Parent material: The organic and inorganic material in which soils form is the parent material, such as bedrock, sand, glacial drift, river sediment.
- Climate: Temperature, moisture, and wind cause different patterns of weathering and therefore affect soil characteristics. The presence of moisture and nutrients from weathering will also promote biological activity.
- Topography: Regional surface features can have a major influence on the characteristics and fertility of a soil. Topography affects water runoff, which strips away parent material and affects plant growth. Steeps soils are more prone to erosion and may be thinner than soils that are relatively flat or level.
- Biological factors: Animals and microorganisms can produce pores and crevices, and plant roots can penetrate into crevices to produce more fragmentation. Leaves and other material that fall from plants will decompose and contribute to soil composition.
- Time: Time is an important factor in soil formation because soils develop over long periods. Soil formation is dynamic: materials are deposited over time, decompose, and transform into other materials that can be used by living organisms or deposited onto the surface of the soil. Depending on the climate, it is estimated to take between 200 to 1000 years to create just 1 inch of soil.
Soil Composition Influences Nutrient Availability to Plants
The information below was adapted from OpenStax Biology 30.3
Even though most plants are autotrophs and can generate their own sugars from carbon dioxide and water, they still require certain ions and minerals from the soil. This process is mediated by root hairs, which are extensions of the root epidermal tissue that increase the surface area of the root, greatly contributing to the absorption of water and minerals. However, the properties of the soil can directly influence the availability of the specific ions present in the soil. The most critical soil property that influences ion availability is the presence (or absence) of clay:
- Clay is negatively charged, and thus any positive ions (cations) that are present in clay-rich soils will remain tightly bound to the clay particles. This tight association with clay particles prevents the cations from being washed away by heavy rains, but it also prevents the cations from being easily absorbed by plant root hairs.
- In contrast, the negatively charged anions are easily dissolved in soil water and thus readily accessible to plant root hairs; however, they are also very easily washed away by rainwater.
As a result of the factors noted above, the presence of large amounts of clay particles in soil present a trade-off for plants: clay particles prevent leaching of cations from the soil by rainwater, but they also prevent absorption of the cations by the plant.
Different soil compositions present other types of tradeoffs for plant roots:
- Sandy soil consist of loosely packed soil particles, meaning there are lots of air pockets that facilitate root penetration and respiration; however, the loose packing also means that water drains away easily – typically taking many nutrients away with it
- Clay soil retains water well as the water molecules remain associated with the charged clay surfaces; however, clay particles pack tightly together meaning that there is less air available in the soil and posing greater difficulty for plant roots to penetrate the dense soil
- Large amounts of organic matter in soil provide a near-ideal environment for plant roots, with high concentrations of nutrients, high water retention, and loose soil packing with many air pockets
The information described above is organized in table form below:

Processes and Structures that Move Molecules into and out of Cells
Before we are able to discuss the details of how plants take up nutrients from the soil, we must first define several structures and process that control movement of molecules across cell membranes:
- Diffusion occurs when molecules move from an area of high concentration to an area of low concentration. Diffusion does not require energy because the molecules move “down” their concentration gradient (from areas of high to low concentration).
- Facilitated diffusion is diffusion that occurs through a protein channel embedded in a cell membrane. Each protein channel is highly specific for its given molecule. Facilitated diffusion is necessary for molecules that are too large or too polar to cross the nonpolar cell membrane.
- Active transport is movement of a molecule against its concentration gradient. Active transport always requires an expenditure of energy, often (but not always) from ATP.
- Proton pumps a proteins complexes that use energy from ATP to “pump” protons from one side of a membrane to other. This process creates an electrochemical gradient, with a high concentration of positively-charged protons on one side of a plasma membrane. This electrochemical gradient can then be used as a source of energy to move other molecules against their concentration gradients via co-transporters.
- Co-transport the movement of two molecules at the same time: one molecule is transported along (“down”) its concentration gradient, which releases energy that is used to transport the other molecule against its concentration gradient. Co-transport occurs through protein channels called co-transporters. As with all protein channels, co-transporters are highly specific for the given molecules that they can transport.
Plant Uptake of Mineral Nutrients from the Soil
How do plants overcome these tradeoffs in order to absorb nutrients from soil water into their root hairs? This process relies upon proton pumps, cation channels, and anion cotransporter channels present in the membranes of the root hairs as follows:
- The epidermal tissue of root hairs is lined by proton pumps (H+ ATPases), which use ATP as an energy source to pump protons out of the cells and into the soils, against their electrochemical gradient. These proton pumps create a strong electrochemical gradient with a high concentration of protons and a strong positive charge outside of the cell, and a low concentration of protons and relatively negative charge inside of the cell. These protons pumped into the soil by the proton pumps cause two direct outcomes:
- The positively-charged protons bind to the negatively-charged clay particles in the soil, releasing the cations from the clay in a process called cation exchange. The cations then diffuse down their electrochemical gradient into the root hairs through cation channels. (The soil environment is highly positively charged, so it is energetically favorable for cations to move into the root hairs and out of the soil environment).
- The high concentration of protons in the soil creates a strong electrochemical gradient that favors transport of protons back into the root hairs. Plants use co-transport of protons down their concentration gradient as the energy source to also move anions against their concentration gradient into the root hairs. This process occurs through anion cotransporter channels. (The soil environment is highly positively charged, so it is energetically unfavorable for anions to leave the soil, but highly energetically favorable for protons to leave the soil).
Watch the videos below for discussion of these interactions, including the importance of pH and clay in soils and the process of cation exchange utilized by plants to acquire nutrients from clay-rich soils.
If the concepts discussed above feel unfamiliar to you, then we recommend the video below for discussion of the principles of active and passive transport (start at 1 min 7 sec and watch through 5 min 14 sec):
Plant Mutualisms with Rhizobia Bacteria and Mycorrhizal Fungi
The information below was adapted from OpenStax Biology 31.3
While plants have ready access to carbon (carbon dioxide) and water (except in dry climates or during drought), nitrogen is often the most limiting nutrient for their growth. Nitrogen is one critical macronutrient necessary for all life, because it is part of nucleic acids and proteins. Atmospheric nitrogen, which is the diatomic molecule N2, comprises approximately 80% of the atmosphere; however, gaseous nitrogen is exceptionally chemically stable and not biologically available to plants because they do not have the necessary enzymes to convert it into biologically useful forms.
There are effectively only two natural sources of biologically available nitrogen for plants: conversion of atmospheric nitrogen into ammonia by specific bacteria species, and the release of nitrogen from biomacromolecules of dead organisms by decomposers such as fungi. Many plants have evolved mutualistic relationships with microorganisms, such as specific species of bacteria and fungi, to enhance their ability to acquire nitrogen and other nutrients from the soil. These relationships are mutually beneficial to both the plant and the microbes.
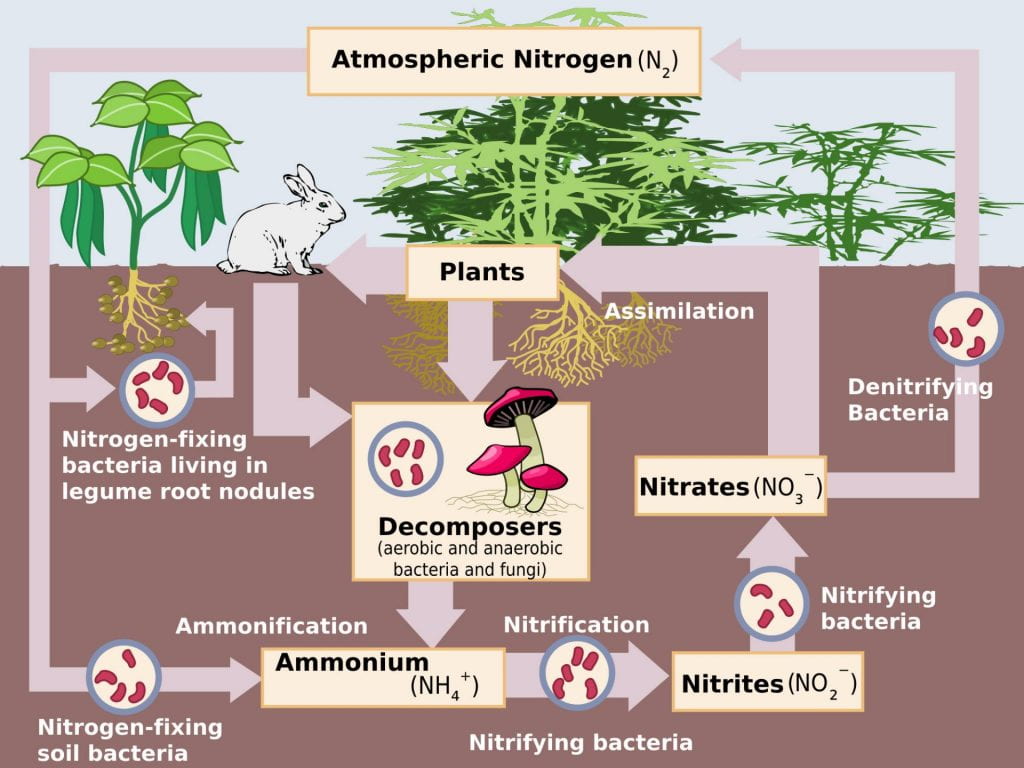
Plant relationships with rhizobia bacteria:
- Legumes (including clover, alfalfa, peanuts, beans, chickpeas, soybeans, and lentils), are capable of mutualistic symbioses with a group of soil bacteria called rhizobia, forming specialized structures in the plant roots called nodules.
- Rhizobia are among the small number of bacterial species that are capable of “fixing” atmospheric nitrogen, or making it biologically available. These bacteria convert atmospheric nitrogen (N2) into ammonia (NH3) within the legume nodules.
- The bacteria use the enzyme nitrogenase to reduce atmospheric nitrogen to ammonia. Importantly, the nitrogenase enzyme is inactivated in the presence of oxygen. The plant root produces a molecule called leghemoglobin, which binds to oxygen to maintain a low oxygen concentration “protect” the rhizobia nitrogenase from oxygen poisoning.
- As in any mutualistic relationships, both organisms benefit from the interaction: the plant obtains ammonia, and bacteria obtain carbon compounds generated through photosynthesis, as well as a protected niche in which to grow.
- The process also contributes to soil fertility, because the plant root system leaves behind some of the biologically available nitrogen.
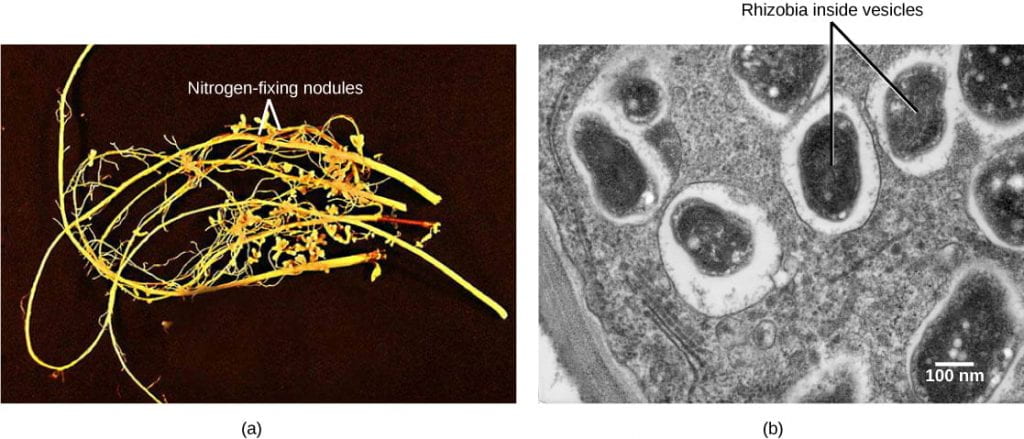
Plant relationships with mycorrhizal fungi:
- It is estimated that ~80-90% rely on mutualistic relationships with mycorrhizal fungi to facilitate the uptake of mineral nutrients from the soil.
- Mycorrhizae integrate the fungi into the physical structure of the plant roots, as the fungi colonize the living root tissue during active plant growth.
- Ectomycorrhizal fungi have hyphae that wrap around the epidermal cells of the root.
- Endomycorrhizal fungi (also called arbuscular fungi) have hyphae that can penetrate the cell walls (though not the cell membranes) of the plant root cells.
- The fungus acquires nitrogen, phosphate, and other minerals by decomposing dead organic matter in the soil and making it biologically available to both itself and its associated plant root; in return, the fungus obtains sugars and other nutrients from the plant root.
- The mycorrhizae also increase the surface area of the plant root system because the fungal hyphae can spread far beyond the plant root system, further maximizing this mutualistic relationship between plant and fungi.
