Learning Objectives
- Explain water potential and predict movement of water in plants by applying the principles of water potential
- Describe the effects of different environmental or soil conditions on the typical water potential gradient in plants
- Identify and differentiate between the three pathways water and minerals can take from the root hair to the vascular tissue
- Explain the three hypotheses explaining water movement in plant xylem, and recognize which hypothesis explains the heights of plants beyond a few meters
- Define transpiration and identify the source of energy that drives transpiration
Water Potential and Water Transport from Roots to Shoots
The information below was adapted from OpenStax Biology 30.5
The structure of plant roots, stems, and leaves facilitates the transport of water, nutrients, and products of photosynthesis throughout the plant. The phloem is the tissue primarily responsible for movement of nutrients and photosynthetic produces, and xylem is the tissue primarily responsible for movement of water). Plants are able to transport water from their roots up to the tips of their tallest shoot through the combination of water potential, evapotranspiration, and stomatal regulation – all without using any cellular energy!
Water potential is a measure of the potential energy in water based on potential water movement between two systems. Water potential can be defined as the difference in potential energy between any given water sample and pure water (at atmospheric pressure and ambient temperature). Water potential is denoted by the Greek letter Ψ (psi) and is expressed in units of pressure (pressure is a form of energy) called megapascals (MPa). The potential of pure water (Ψpure H2O) is defined as zero (even though pure water contains plenty of potential energy, this energy is ignored in this context).
Water potential can be positive or negative, and water potential is calculated from the combined effects of solute concentration (s) and pressure (p). The equation for this calculation is Ψsystem = Ψs + Ψp, where Ψs = solute potential, and Ψp = pressure potential.
Adding more dissolved solutes will decrease the water potential, and removing dissolved solutes will increase the water potential. Adding more pressure (positive pressure) will increase the water potential, and removing pressure (including creating a vacuum, or negative pressure) will decrease the water potential.
Water always moves from a region of high water potential to an area of low water potential, until it equilibrates the water potential of the system. At equilibrium, there is no difference in water potential on either side of the system (the difference in water potentials is zero). This means that the water potential at a plant’s roots must be higher than the water potential in each leaf, and the water potential in the plant’s leaves must be higher than the water potential in the atmosphere, in order for water to continuously move through the plant from the soil to the air without equilibrating (a process called transpiration). Mathematically: Ψsoil must be > Ψroot > Ψstem > Ψleaf > Ψatmosphere.
Now let’s consider solute and pressure potential in the context of a plant’s cells:
- The solute potential (Ψs), also called osmotic potential, of pure water is 0. Recall that dissolving more solutes in a water sample results in decreased water potential; thus the solute potential of a plant cell is negative, because of the high solute concentration of the cell cytoplasm. As long as the water potential in the plant root cells is lower than the water potential of the water in the soil, then water will move from the soil into a plant’s root cells via osmosis. In fact, plant cells can metabolically manipulate Ψs by adding or removing solute molecules to increase water uptake from the soil during drought conditions.
- Pressure potential (Ψp), also called turgor potential, may be positive or negative. Positive pressure (compression) increases Ψp, and negative pressure (vacuum) decreases Ψp. Positive pressure inside cells is contained by the rigid cell wall, producing turgor pressure. Pressure potentials can reach as high as 1.5 MPa in a well-watered plant. A Ψp of 1.5 MPa equates to 210 pounds per square inch (psi); for a comparison, most automobile tires are kept at a pressure of 30-34 psi. Plant cells can indirectly manipulate Ψp via their ability to directly manipulate Ψs and by the process of osmosis: if a plant cell increases the cytoplasmic solute concentration, then Ψs will decline and water will move into the cell by osmosis, causing Ψp to increase. Ψp is also under indirect plant control via the opening and closing of stomata; stomatal openings allow water to evaporate from the leaf, reducing Ψp and Ψtotal of the leaf and increasing the water potential difference between the water in the leaf and the petiole, thereby allowing water to flow from the petiole into the leaf.
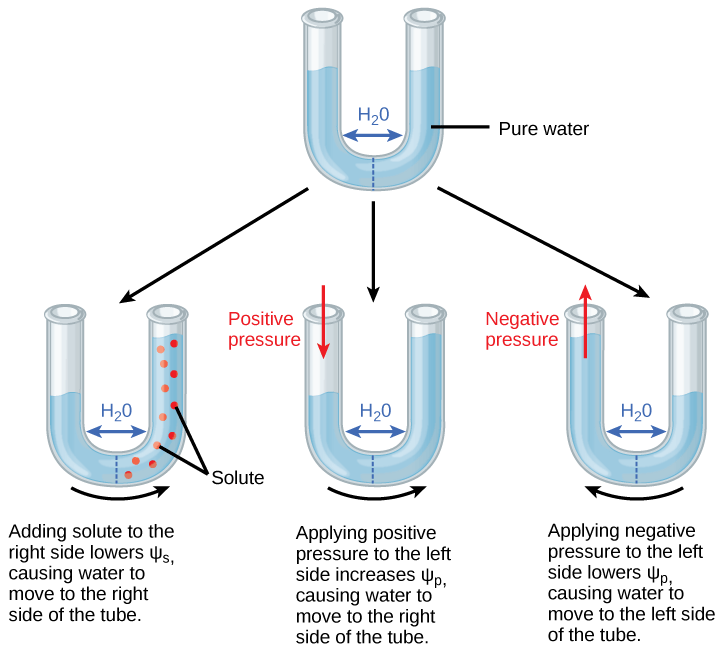
An example of the effect of turgor pressure is the wilting of leaves and their restoration after the plant has been watered. Water is lost from the leaves via transpiration (approaching Ψp = 0 MPa at the wilting point) and restored by uptake via the roots.
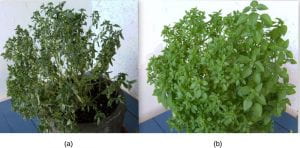
This video provides an overview of water potential, including solute and pressure potential (stop after 5:05):
And this video describes how plants manipulate water potential to absorb water and how water and minerals move through the root tissues:
Impact of Soil and Environmental Conditions on the Plant Water Potential Gradient
As noted above, Ψsoil must be > Ψroot > Ψstem > Ψleaf > Ψatmosphere in order for transpiration to occur (continuous movement of water through the plant from the soil to the air without equilibrating. This continuous movement of water relies on a water potential gradient, where water potential decreases at each point from soil to atmosphere as it passes through the plant tissues. However, this gradient can become disrupted if the soil becomes too dry, which can result in both decreased solute potential (due to the same amount of solutes dissolved in a smaller quantity of water) as well as decreased pressure potential in severe droughts (resulting from negative pressure or a “vacuum” in the soil due to loss of water volume). If water potential becomes sufficiently lower in the soil than in the plant’s roots, then water will move out of the plant root and into the soil.
Pathways of Water and Mineral Movement in the Roots
The information below was adapted from OpenStax Biology 30.5
Once water has been absorbed by a root hair, it moves through the ground tissue and along its water potential gradient through one of three possible routes before entering the plant’s xylem:
- the symplast: “sym” means “same” or “shared,” so symplast is “shared cytoplasm”. In this pathway, water and minerals move from the cytoplasm of one cell in to the next, via plasmodesmata that physically join different plant cells, until eventually reaching the xylem.
- the transmembrane pathway: in this pathway, water moves through water channels present in the plant cell plasma membranes, from one cell to the next, until eventually reaching the xylem.
- the apoplast: “a” means “outside of,” so apoplast is “outside of the cell”. In this pathway, water and dissolved minerals never move through a cell’s plasma membrane but instead travel through the porous cell walls that surround plant cells.
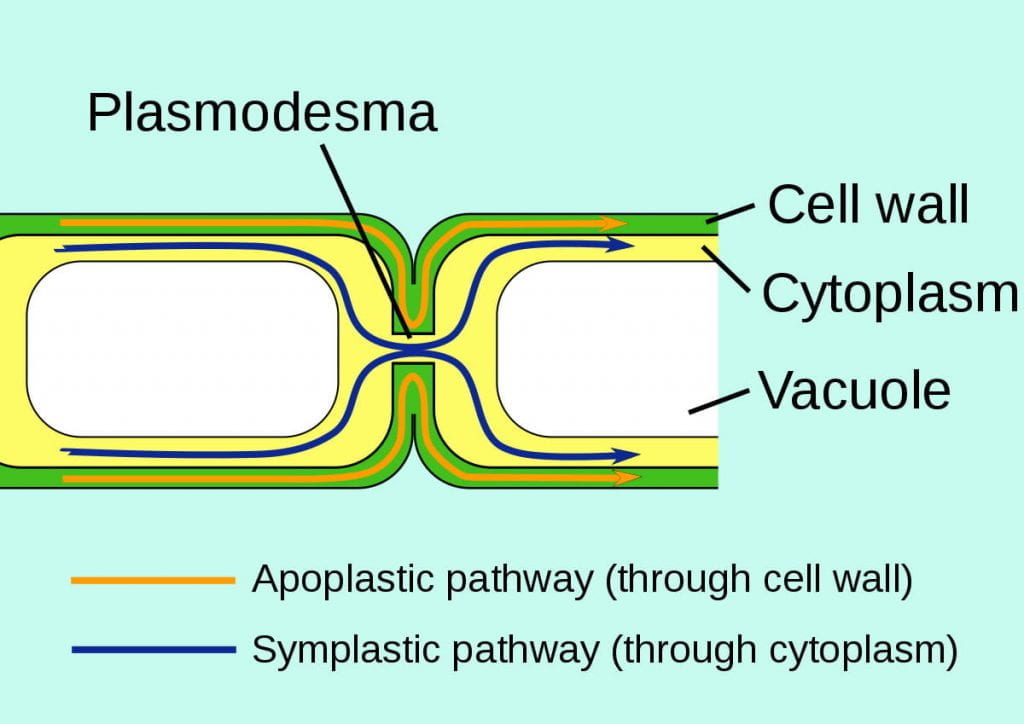
Water and minerals that move into a cell through the plasma membrane has been “filtered” as it passes through water or other channels within the plasma membrane; however water and minerals that move via the apoplast do not encounter a filtering step until they reach a layer of cells known as the endodermis which separate the vascular tissue (called the stele in the root) from the ground tissue in the outer portion of the root. The endodermis is present only in roots, and serves as a checkpoint for materials entering the root’s vascular system. A waxy substance called suberin is present on the walls of the endodermal cells. This waxy region, known as the Casparian strip, forces water and solutes to cross the plasma membranes of endodermal cells instead of slipping between the cells. This process ensures that only materials required by the root pass through the endodermis, while toxic substances and pathogens are generally excluded.
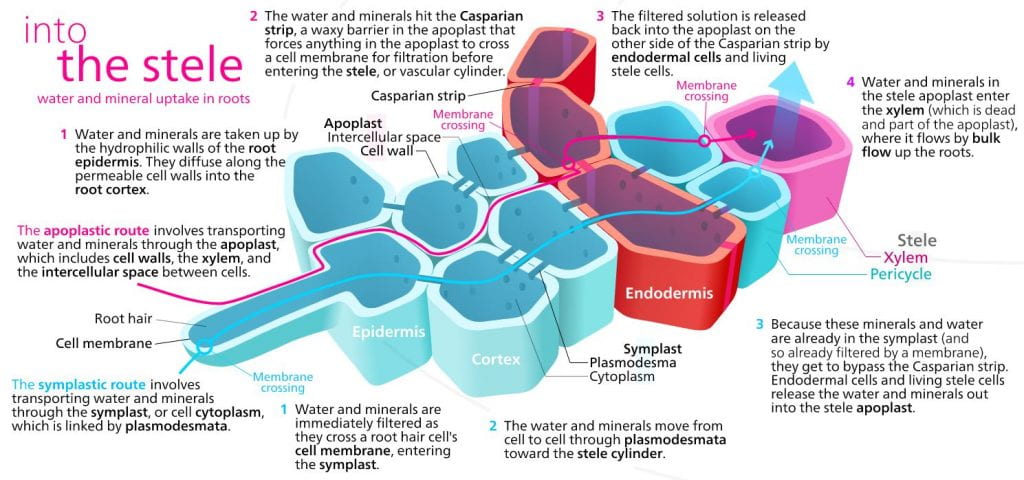
Movement of Water Up the Xylem Against Gravity
How is water transported up a plant against gravity, when there is no “pump” or input of cellular energy to move water through a plant’s vascular tissue? There are three hypotheses that explain the movement of water up a plant against gravity. These hypotheses are not mutually exclusive, and each contribute to movement of water in a plant, but only one can explain the height of tall trees:
- Root pressure pushes water up
- Capillary action draws water up within the xylem
- Cohesion-tension pulls water up the xylem
We’ll consider each of these in turn.
Root pressure relies on positive pressure that forms in the roots as water moves into the roots from the soil. Water moves into the roots from the soil by osmosis, due to the low solute potential in the roots (lower Ψs in roots than in soil). This intake of water in the roots increases Ψp in the root xylem, “pushing” water up. In extreme circumstances, or when stomata are closed at night preventing water from evaporating from the leaves, root pressure results in guttation, or secretion of water droplets from stomata in the leaves. However, root pressure can only move water against gravity by a few meters, so it is not sufficient to move water up the height of a tall tree.
Capillary action (or capillarity) is the tendency of a liquid to move up against gravity when confined within a narrow tube (capillary). You can directly observe the effects of capillary action when water forms a meniscus when confined in a narrow tube. Capillarity occurs due to three properties of water:
- Surface tension, which occurs because hydrogen bonding between water molecules is stronger at the air-water interface than among molecules within the water.
- Adhesion, which is molecular attraction between “unlike” molecules. In the case of xylem, adhesion occurs between water molecules and the molecules of the xylem cell walls.
- Cohesion, which is molecular attraction between “like” molecules. In water, cohesion occurs due to hydrogen bonding between water molecules.
On its own, capillarity can work well within a vertical stem for up to approximately 1 meter, so it is not strong enough to move water up a tall tree.
This video provides an overview of the important properties of water that facilitate this movement:
The cohesion-tension hypothesis is the most widely-accepted model for movement of water in vascular plants. Cohesion-tension combines the process of capillary action with transpiration or the evaporation of water from the plant stomata. Transpiration is ultimately the main driver of water movement in xylem, combined with the effects of capillary action. The cohesion-tension model works like this:
- Transpiration (evaporation) occurs because stomata in the leaves are open to allow gas exchange for photosynthesis. As transpiration occurs, evaporation of water deepens the meniscus of water in the leaf, creating negative pressure (also called tension or suction).
- The tension created by transpiration “pulls” water in the plant xylem, drawing the water upward in much the same way that you draw water upward when you suck on a straw.
- Cohesion (water molecules sticking to other water molecules) causes more water molecules to fill the gap in the xylem as the top-most water is pulled toward end of the meniscus within the stomata.
Transpiration results in a phenomenal amount of negative pressure within the xylem vessels and tracheids, which are structurally reinforced with lignin to cope with large changes in pressure. The taller the tree, the greater the tension forces (and thus negative pressure) needed to pull water up from roots to shoots.

Follow this link to watch this video on YouTube for an overview of the different processes that cause water to move throughout a plant (this video is linked because it cannot be directly embedded within the textbook; if needed, the video url is https://www.youtube.com/watch?v=8YlGyb0WqUw)
Transpiration Energy Source
The term “transpiration” has been used throughout this reading in the context of water movement in plants. Here we will define it as: evaporation of water from the plant stomata resulting in the continuous movement of water through a plant via the xylem, from soil to air, without equilibrating.
Transpiration is a passive process with respect to the plant, meaning that ATP is not required to move water up the plant’s shoots. The energy source that drives the process of transpiration is the extreme difference in water potential between the water in the soil and the water in the atmosphere. Factors that alter this extreme difference in water potential can also alter the rate of transpiration in the plant.