Learning Objectives
- Differentiate between neurons, nerves, and nervous systems
- Describe different types of nervous systems in different animal lineages
- Identify, differentiate between, and describe basic structures and functions of the central versus peripheral nervous systems
- Define learning and memory, and explain the roles of the hippocampus, the cerebral cortex, neuroplasticity, and synaptic plasticity in learning and memory
- Describe the roles of and differentiate between the divisions of the vertebrate nervous system (afferent, efferent, somatic, autonomic, sympathetic, parasympathetic)
- Recognize the features of a reflex arc, and use reflex arcs as a model to identify the roles of different components of the nervous system
Neurons, Nerves, and Nervous Systems
The information below was adapted from OpenStax Biology 35.0 and Khan Academy Overview of neuron structure and function. All Khan Academy content is available for free at www.khanacademy.org
A nervous system is an organism’s control center: a network of neurons which send communications between and across different parts of the body; a nervous system collects and processes sensory information from outside (and inside) the body and controls all behaviors, from eating to sleeping to finding a mate.
The nervous system is made up of neurons, specialized cells that can receive and transmit chemical or electrical signals, and glia, cells that provide support functions for the neurons by playing an information processing role that is complementary to neurons. Nerves are bundles of nervous tissue, often containing hundreds to thousands of axons from different neurons, wrapped in connective tissue.
Diversity of Nervous Systems
The information below was adapted from OpenStax Biology 35.1
Nervous systems throughout the animal kingdom vary in structure and complexity:
- All animals have a true nervous system except sea sponges.
- Cnidarians, such as jellyfish, lack a true brain but have a system of separate but connected neurons called a nerve net.
- Echinoderms, such as sea stars, have neurons that are bundled into nerves.
- Flatworms of the phylum Platyhelminthes have both a central nervous system (CNS) made up of a small brain and two nerve cords, and peripheral nervous system (PNS) containing a system of nerves that extend throughout the body.
- The insect nervous system is more complex but also fairly decentralized, with a brain, ventral nerve cord, and ganglia (clusters of connected neurons); these ganglia can control movements and behaviors without input from the brain.
- Cephalopods, such as octopi, may have the most complex nervous systems among invertebrates, with neurons that are organized in specialized lobes and eyes that are structurally similar to vertebrate species.
- Compared to invertebrates, vertebrate nervous systems are more complex, centralized, and specialized. While there is great diversity among different vertebrate nervous systems, they all share a basic structure: a CNS that contains a brain and spinal cord and a PNS made up of peripheral sensory and motor nerves.
One interesting difference between the nervous systems of invertebrates and vertebrates is that the nerve cords of many invertebrates are located ventrally (along the belly) whereas the vertebrate spinal cords are located dorsally (along the back). There is debate among evolutionary biologists as to whether these different nervous system plans evolved separately or whether the original invertebrate body plan arrangement somehow “flipped” during the evolution of vertebrates.

The Nervous System in Vertebrates
The information below was adapted from OpenStax Biology 35.3 and Khan Academy Overview of neuron structure and function. All Khan Academy content is available for free at www.khanacademy.org
For the rest of this reading, we’ll focus on the vertebrate nervous system. In vertebrates, the nervous system can be broadly divided into two sections:
- a central nervous system (CNS) consisting of:
- the brain, a structure that processes information, composed of inter-connected neurons and glial cells
- the spinal cord, a structure that transmits information, consisting of a thick bundle of nerve tissue that carries information about the body to the brain and from the brain to the body
- both the brain and the spinal cord contain interneurons, which are found only in the CNS. Interneurons receive information from one set of neurons (usually afferent – see below) and transmit information to another set of neurons (usually efferent – see below)
- a peripheral nervous system (PNS) that collects information and sends commands, containing nerves that extend to and from the spinal cord and are divided into:
- afferent nerves (also called sensory nerves) which are composed of afferent neurons (also called sensory neurons) that collect sensory information from the body and transmit it to the CNS
- efferent nerves (also called motor nerves) which are composed of efferent neurons (also called motor neurons) that carry commands from the CNS to the body
The material in the nervous system can also be classified based on whether it contains white matter (myelinated axons) and gray matter (unmyelinated axons and cell bodies).

CNS Part I: the Brain
The brain is the part of the central nervous system that is contained in the skull. It floats in a fluid called cerebrospinal fluid (CSF), which is filtered from arterial blood. CSF acts as a cushion and shock absorber and makes the brain neutrally buoyant. Specialized glial cells help circulate the CSF to distribute and exchange chemical substances throughout the brain and into the spinal cord. These glial cells line the fluid-filled cavities in the center of the brain, called ventricles, which also contains CSF.
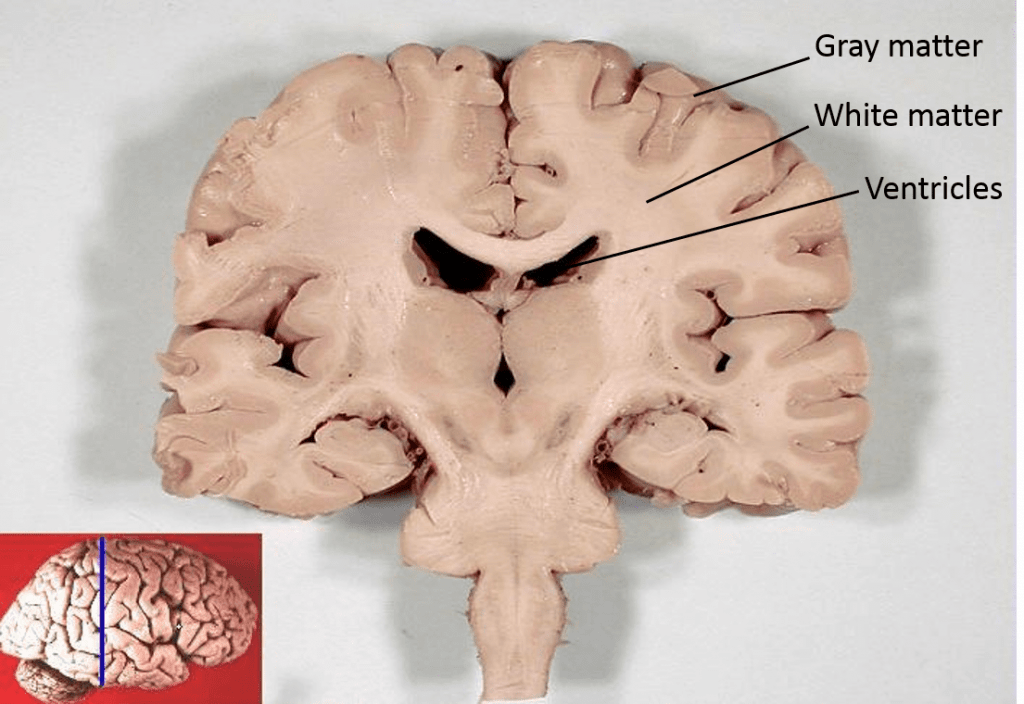
At the general anatomy level (shown above), the brain is organized with white matter (myelinated axons) toward the inside of the brain, and gray matter (unmyelinated axons and cell bodies) on the outside of the brain:
- Gray matter represents the information-processing centers of the brain
- White matter represents the networking between these processing centers
These white gray matter processing centers include (but are not limited to):
- The cerebrum and cerebral cortex make up the majority of the human brain. The outermost part of the cerebrum is called the cerebral cortex, which is folded into “hills” and “valleys.”
- The cortex is made up of two hemispheres (right and left) and four lobes (frontal, parietal, temporal, occipital).
- The two hemispheres are joined by a thick fiber bundle called the corpus callosum (Latin: “tough body”) which connects the two hemispheres and allows information to be passed from one side to the other.
- Although there are some brain functions that are localized more to one hemisphere than the other, the functions of the two hemispheres are largely redundant.
- The diencephalon controls homeostasis and acts as a relay station, transmitting sensory information from sensory neurons to the cerebrum. The structures located in the diencephalon that regulate these processes include the thalamus and the hypothalamus.
- The thalamus acts as a gateway to and from the cortex.; it receives sensory and motor inputs from the body and also receives feedback from the cortex.
- Below the thalamus is the hypothalamus, which controls the endocrine system by sending signals to the pituitary gland, the “master regulator” of the endocrine system. This relationship means that the hypothalamus regulates important behaviors that are controlled by these hormones. The hypothalamus is effectively the body’s “thermostat” (think negative feedback loop); it makes sure key functions like food and water intake, energy expenditure, and body temperature are kept at appropriate levels.
- The cerebellum (Latin for “little brain”) sits at the base of the brain on top of the brainstem. The cerebellum controls balance and aids in coordinating complex movements and learning new motor tasks.
- The brainstem connects the rest of the brain with the spinal cord. Motor and sensory neurons extend through the brainstem allowing for the relay of signals between the brain and spinal cord. The brainstem controls several important functions of the body that do not require conscious thought, including alertness, arousal, breathing, blood pressure, digestion, heart rate, swallowing, walking, and sensory and motor information integration.
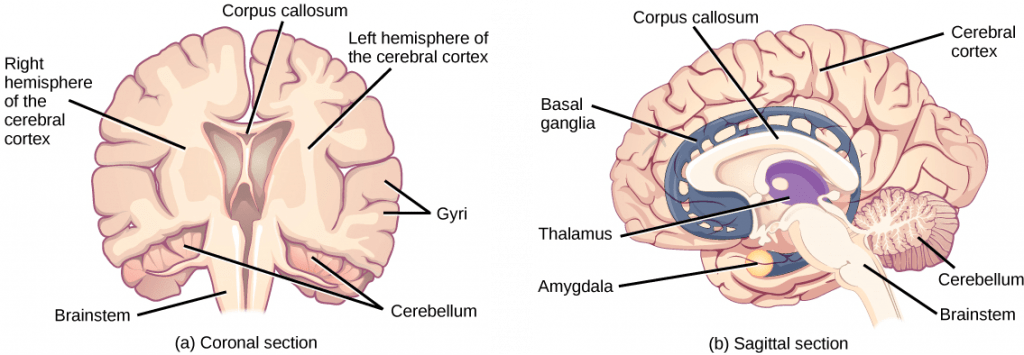
Each hemisphere of the cerebrum contains regions called “lobes” that are involved in different functions:
- The frontal lobe is located at the front of the brain, over the eyes. This lobe contains the olfactory bulb, which processes smells, and the motor cortex, which is important for planning and implementing movement. Neurons in the frontal lobe also control cognitive functions like maintaining attention, speech, and decision-making, and parts of this area are involved in personality, socialization, and assessing risk.
- The parietal lobe is located at the top of the brain. Neurons in the parietal lobe are involved in speech and also reading, as well as processing somatosensation (touch sensations like pressure, pain, heat, cold) and processing proprioception (the sense of how parts of the body are oriented in space).
- The occipital lobe is located at the back of the brain. It is primarily involved in vision: seeing, recognizing, and identifying the visual world. The occipital nerve transmits sensory information from the eyes at the front of the skull to the occipital lobe at the back of the brain.
- The temporal lobe is located at the base of the brain by your ears and is primarily involved in processing and interpreting sounds. It also contains the hippocampus, which processes memory formation and is critical for learning (more on learning and memory below).
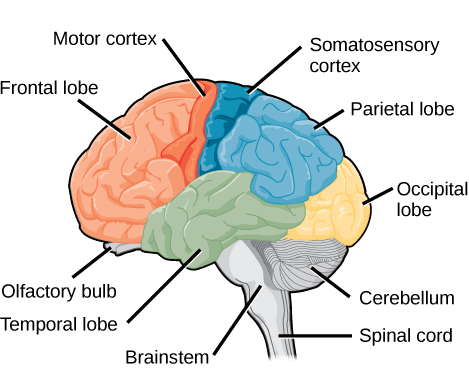
Compared to other vertebrates, mammals have very large brains for their body size. An entire alligator’s brain, for example, would fill about one and a half teaspoons. This increase in brain to body size ratio is especially pronounced in apes, whales, and dolphins. In addition, scientists have found a relationship between the relatively high surface area of the cortex and both the intelligence and the complex social behaviors exhibited by some mammals. This increased surface area is due, in part, to increased folding (“hills and valleys”) of the frontal cortex; a rat cortex has less folding than a cat cortex, which has less folding than a primate or dolphin cortex.
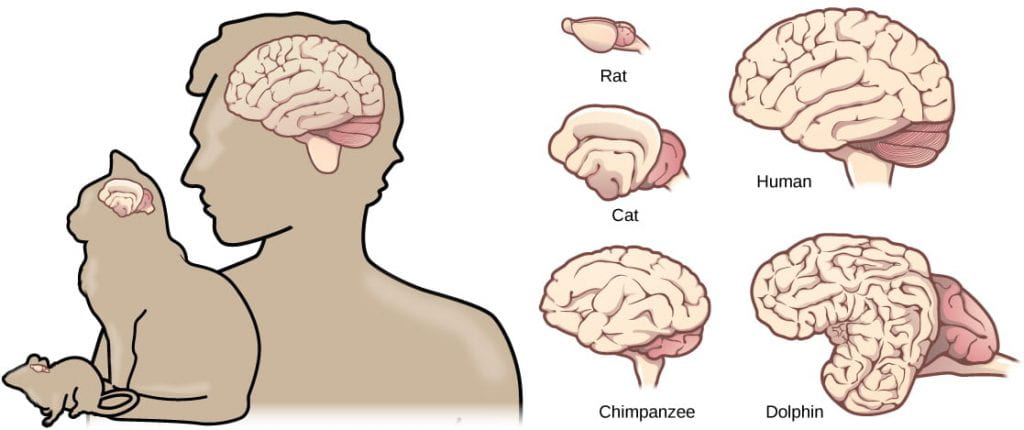
This video provides a summary of the functions of different parts of the vertebrate brain (watch the first four minutes):
Learning and Memory
The information below was adapted from OpenStax Biology 35.2
One of the key functions that the brain performs is the process of learning and memory. Learning is the ability to acquire new knowledge, and memory is the ability to recall it later. Learning and memory involve both specific brain structures as well as certain neuronal processes. The current hypothesis states that specific neurons in the cerebral cortex are responsible for physically storing memories, and that learning and memory are mediated by both chemical and structural changes in the synapses of these neurons. This process of the formation of new and loss of existing neurons is called neural plasticity.
- Brain structures involved in learning and memory:
- Short-term memories are thought to be stored in the prefrontal cortex (part of the frontal lobe of the cerebral cortex).
- The hippocampus in the temporal lobe is essential for consolidating these short-term memories into long-term memories, but the memories are not actually stored in the hippocampus.
- The precise location of memory storage is unknown, but it is thought that different components of memories may be stored in different locations within the cerebral cortex, and that retrieval of long-term memories may involve the prefrontal cortex.
- Chemical and structural changes in synapses involved in learning and memory:
- Neurogenesis, or the growth of new neurons. At one time, scientists believed that people were born with all the neurons they would ever have, but research performed during the last few decades indicates that neurogenesis, the birth of new neurons, continues into adulthood. Neurogenesis was first discovered in songbirds that produce new neurons while learning songs. For mammals, new neurons also play an important role in learning: about 1000 new neurons develop in the hippocampus (a brain structure involved in learning and memory) each day. While most of the new neurons will die, researchers found that an increase in the number of surviving new neurons in the hippocampus correlated with how well rats learned a new task. Interestingly, both exercise and some antidepressant medications also promote neurogenesis in the hippocampus. Stress has the opposite effect.
- Synaptogenesis, or the growth of new synapses between two existing neurons, and synaptic pruning, or the destruction of existing synapses between two neurons.
- Synaptic plasticity, or the strengthening or weakening of existing synaptic connections. Two processes in particular, long-term potentiation (LTP) and long-term depression (LTD) are important forms of synaptic plasticity that occur in synapses in the hippocampus, a brain region that is involved in storing memories.
- Long-term potentiation (LTP) is the long-term strengthening of a synaptic connection. LTP is based on the idea that “cells that fire together wire together.” There are various mechanisms underlying synaptic strengthening seen with LTP, including an increase in the amount of neurotransmitter released by the presynaptic neuron, and an increased response to the same amount of neurotransmitter by the postsynaptic neuron. LTP can result in sensitization, where there is an increased response to the same external stimulus.
- Long-term depression (LTD) is essentially the reverse of LTP: it is a long-term weakening of a synaptic connection. While it may seem counterintuitive, LTD may be just as important for learning and memory as LTP: the weakening unused synapses allows for unimportant connections to be lost and makes the synapses that have undergone LTP that much stronger by comparison. LTD can result in habituation, where there is a decreased response to the same external stimulus.
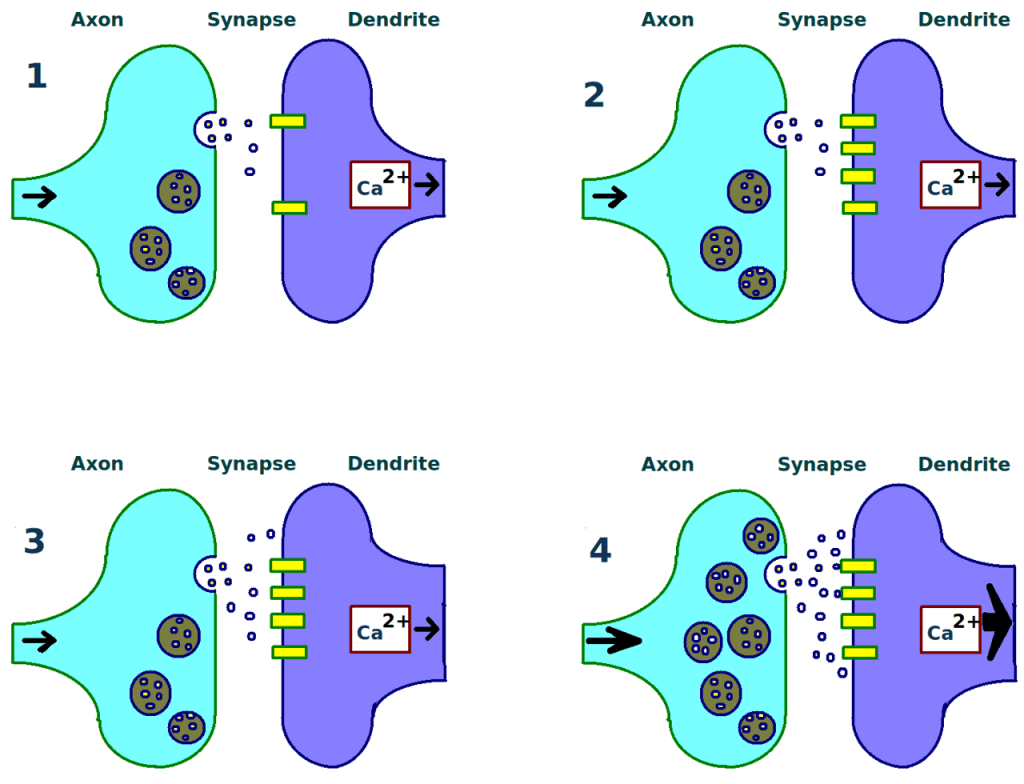
This video provides a simplified overview of learning and memory in a commonly used model organism for studying these processes:
And finally, this video provides a succinct overview of two of the common results of learning, sensitization or habituation:
CNS Part II: the Spinal Cord
The information below was adapted from OpenStax Biology 35.3 and Khan Academy Overview of neuron structure and function. All Khan Academy content is available for free at www.khanacademy.org
In order to learn from and respond to information, the brain first must receive that information from the environment. Detection of information occurs via the PNS, and the information is relayed from the PNS to the CNS: the spinal cord and the brain. The spinal cord is a thick bundle of nerve tissue that connects to the brainstem and extends down the body through the spinal column; the spinal cord is contained within the bones of the vertebrate column but is able to communicate signals to and from the body through its connections with spinal nerves (the spinal nerves are part of the PNS, while the spinal column is part of the CNS).
A cross-section of the spinal cord looks like a white oval containing a gray butterfly-shape. Myelinated axons make up the white matter and neuron and glial cell bodies make up the gray matter. Gray matter is also composed of interneurons, which connect two neurons each located in different parts of the body. Relay of information to and from the spinal column is directional:
- Axons and cell bodies in the dorsal spinal cord (facing the back of the animal) convey mostly sensory information from the body to the spinal cord then brain.
- Axons and cell bodies in the ventral spinal cord (facing the belly of the animal) primarily transmit signals controlling movement from the brain to out to the body.
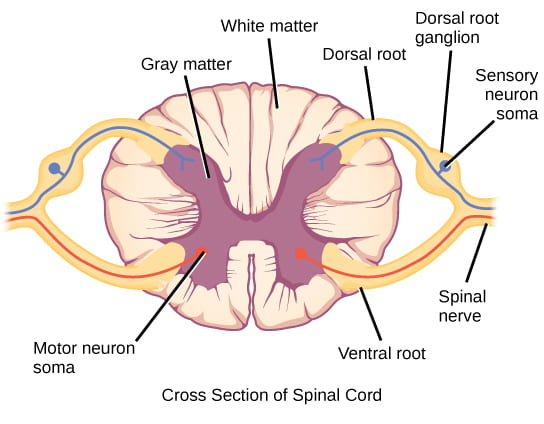
As noted previously, a nerve is not the same thing as a neuron; nerves are actually bundles of neuronal axons, and a nerve can contain hundreds to thousands of axons. Nerves contain both neuronal cells (including axons and associated cells providing myelination) and connective tissue (blood vessels and multiple layers of membranes bundling different groups of axons). The diagram below illustrates the general anatomy of a nerve:

The Peripheral Nervous System in Vertebrates
The information below was adapted from OpenStax Biology 35.4
The spinal column transmits information to the CNS (brain + spinal cord) for processing. How does the information get to the spinal cord to begin with? And how do commands from the CNS get carried out? That is the job of nerves and the peripheral nervous system (PNS). The PNS is composed of the cranial and spinal nerves, and can be divided into the different divisions based on structure and function as follow:
- The afferent (sensory) division: collects incoming sensory information; made up of cranial and spinal nerves that contain sensory neurons. Sensory neurons transmit sensory information from the skin, skeletal muscle, and sensory organs to the CNS. Without its afferent nervous system, an animal would be unable to receive or process any information about its environment (what it sees, feels, hears, and so on).
- The efferent (motor) division: carries outgoing commands; made up of cranial and spinal nerves that contain motor neurons. Motor neurons transmit messages about desired movement from the CNS to the muscles to make them contract. Motor neurons may be under conscious or unconscious control. The efferent division can be further divided into:
- The somatic (conscious control) nervous system: sends motor commands from the CNS to voluntarily-controlled muscles; made up of cranial and spinal nerves that contain motor neurons under conscious control. Without the somatic nervous system, an animal would be unable to respond to its environment via controlled motor movements. Motor neurons of the somatic nervous system synapse with muscles under voluntary control, such as limb muscles.
- The autonomic (unconscious control) nervous system, which controls bodily functions without conscious control; made of cranial and spinal nerves that contain motor neurons under unconscious control, such as the heart, smooth muscle, and exocrine and endocrine glands. Can be further divided into two systems with opposing effects:
- The sympathetic nervous system, which controls the “fight or flight” reactions associated with the short-term stress response. Examples of functions controlled by the sympathetic nervous system include an accelerated heart rate and inhibited digestion. These functions help prepare an organism’s body for the physical strain required to escape a potentially dangerous situation or to fend off a predator. The sympathetic nervous system alters the behavior of many organs directly via synapsed neurons, including the adrenal glands which then release norepinephrine and epinephrine into the blood stream. These hormones then cause further changes, including dilation of the trachea and bronchi (making it easier for the animal to breathe), increasing heart rate, and moving blood from the skin to the heart, muscles, and brain (so the animal can think and run).
- The parasympathetic nervous system: controls the “rest and digest” activities involved in conserving and restoring energy. The parasympathetic nervous system resets organ function after the sympathetic nervous system is activated (the common adrenaline dump you feel after a “fight-or-flight” event). Effects of the parasympathetic nervous system on target organs include slowing of heart rate, lowered blood pressure, and stimulation of digestion.
The opposing effects of the sympathetic vs parasympathetic nervous systems are illustrated below:
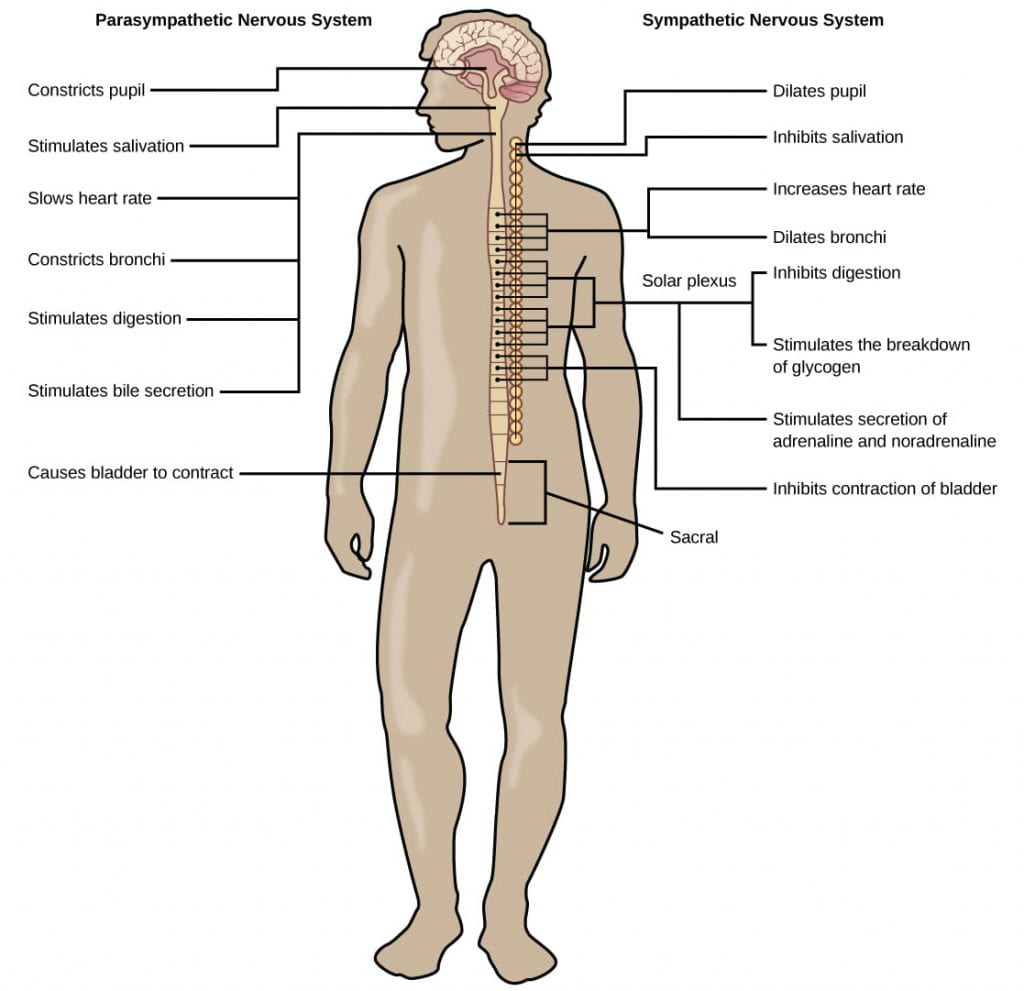
This video provides an overview of the CNS and PNS:
Reflex Arcs
Reflex arcs are an interesting phenomenon for considering how the PNS and CNS work together. Reflexes are quick, unconscious movements, like automatically removing a hand from a hot object, before your brain even detects the heat. Reflexes are so fast because they involve local synaptic connections in the spinal cord, rather than requiring relay of information to the brain. For example, the knee reflex that a doctor tests during a routine physical is controlled by a single synapse between a sensory neuron and a motor neuron. While a reflex may only require the involvement of one or two synapses, synapses with interneurons in the spinal column transmit information to the brain to convey what happened after the event is already over (the knee jerked, or the hand was hot). So this means that the brain is not involved at all in the movement associated with the reflex, but it is certainly involved in learning from the experience – most people only have to touch a hot stove once to learn that they should never do it again!
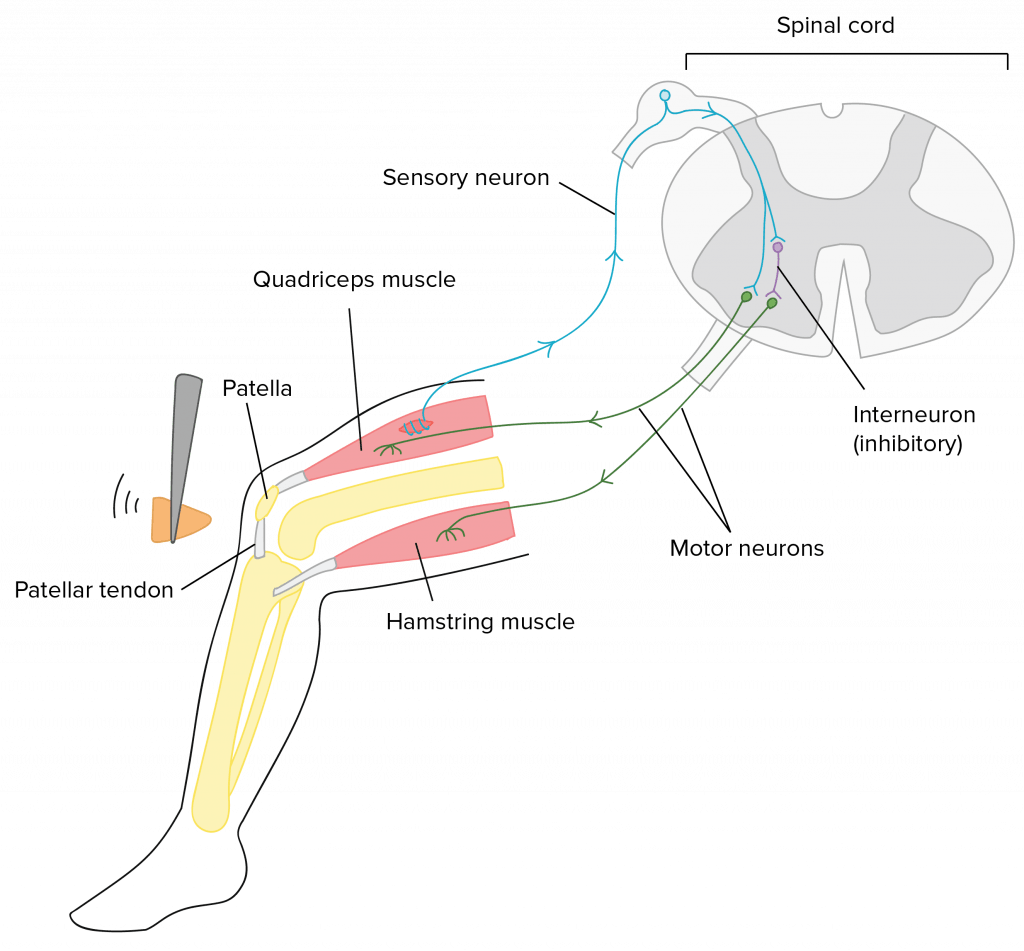
This video provides an overview of how reflex arcs work: