Learning Objectives
- Distinguish between endothermic, ectothermic, homeothermic, heterothermic, and poikilothermic mechanisms of thermoregulation in animals
- Identify and describe the mechanisms that animals use to regulate heat loss and heat gain, including: circulatory adaptations, metabolic activity, insulation, torpor, and behavioral adaptations exploiting the processes of conduction, convection, radiation, and evaporation.
- Identify and describe the mechanisms used by plants to tolerate temporary drought and flooding
- Distinguish between and describe the photosynthetic adaptations to hot and dry conditions present in C4 and CAM plants
Living Things Respond to their Environments
As we have discussed throughout this course, living things can either regulate or conform in response to changes in the environment. Regulating means using internal control mechanisms to minimize internal physiological changes. Conforming allows certain internal conditions to vary with external changes. Here we discuss thermoregulation in animals and responses to water stress and heat in plants as example responses to environmental challenges among living things.
Thermoregulation in Animals
The information below was adapted from OpenStax Biology 33.3
Body temperature affects body activities and function. Generally, as body temperature rises, enzyme activity increases as well. For every ten-degree centigrade rise in temperature, enzyme activity doubles, up to a point: body proteins, including enzymes, begin to denature and lose their function with high heat (around 50oC for mammals). Enzyme activity will decrease by half for every ten-degree centigrade drop in temperature, to the point of freezing, with a few exceptions. Some fish and amphibians can withstand freezing solid and return to normal with thawing.
We can categorize animals according to two dimensions for how they thermoregulate: the animal’s source of heat and the animal’s mechanism of regulation of its body temperature.
Source of body heat:
- Endotherms obtain heat from metabolic activity within their own cells (“endo” = “inside”). Endotherms typically have high metabolic rates (and thus high nutritional needs), and any given endothermic species generally has a relatively narrow range of temperatures in which it can survive. Endothermic animals include mammals and birds.
- Ectotherms obtain heat from external sources (“ecto” = “outside”). Ectotherms typically have lower metabolic rates (and thus lower nutritional needs), and any given ectothermic species generally has a wider range of temperatures in which it can survive compared to an endothermic species. Ectotherms include most fish, amphibians, reptiles, and invertebrates.
Mechanism of body temperature regulation:
- Homeotherms maintain a relatively constant body temperature, even as the external temperature varies (“homeo” = “same”). Note that homeotherms may be endothermic or ectothermic. Most birds and mammals are homeothermic endotherms, and most polar marine fish and invertebrates are homeothermic ectotherms.
- Poikilotherms allow the body temperature to fluctuate with the environment, and include most amphibians, reptiles, freshwater fish, and terrestrial and freshwater invertebrates. Poikilotherms are typically also ectotherms.
- Heterotherms can switch between homoeothermic and poikilothermic strategies; they usually maintain a relatively constant body temperature, but they may have specific periods of day or year where the body temperature fluctuates with the environment. In these cases, the animal enters a seasonal or daily “torpor,” or period of inactivity when they have decreased metabolic activity; winter torpor is also called hibernation. Heterotherms include animals that hibernate (many mammals, some reptiles) or exhibit summer or even daily torpor (some mammals and birds). Mammals that hibernate are heterothermic endotherms, and reptiles that hibernate are heterothermic ectotherms.
These mechanisms of thermoregulation present tradeoffs between the extremes: endotherms and homeotherms have much higher metabolic rates, and require much more food per day, compared to ectotherms and poikilotherms. For example, small mammals and birds that maintain an internal body temperature can spend up to 100 times more energy to thermoregulate in a low temperatures compared to a similarly-sized ectotherm.
This video provides an overview of “cold blooded” vs “warm blooded” animals; note these terms are oversimplifications and we will avoid them in this course:
Regulation of Heat Loss and Heat Gain
The information below was adapted from OpenStax Biology 33.3
All animals exchange heat with their environment, which can significantly impact the animals’ ability to thermoregulate. Heat can be exchanged between an animal and its environment through four mechanisms: radiation, evaporation, convection, and conduction:
- Radiation is the transfer of heat between two bodies that are not in direct contact, via the emission of electromagnetic “heat” waves. Heat comes from the sun in this manner and radiates from dry skin the same way.
- Evaporation is the loss of heat associated with a phase change from liquid to gas. Heat can be removed with liquid from a surface during evaporation, as occurs when a mammal sweats.
- Convection is the transfer of heat between a body and a moving gas or liquid, such as wind or a stream; wind removes heat from the surface of dry skin as the air passes over it.
- Conduction is the transfer of heat between two bodies in direct contact. Heat will be conducted from one surface to another during direct contact with the surfaces, such as an animal resting on a warm rock.
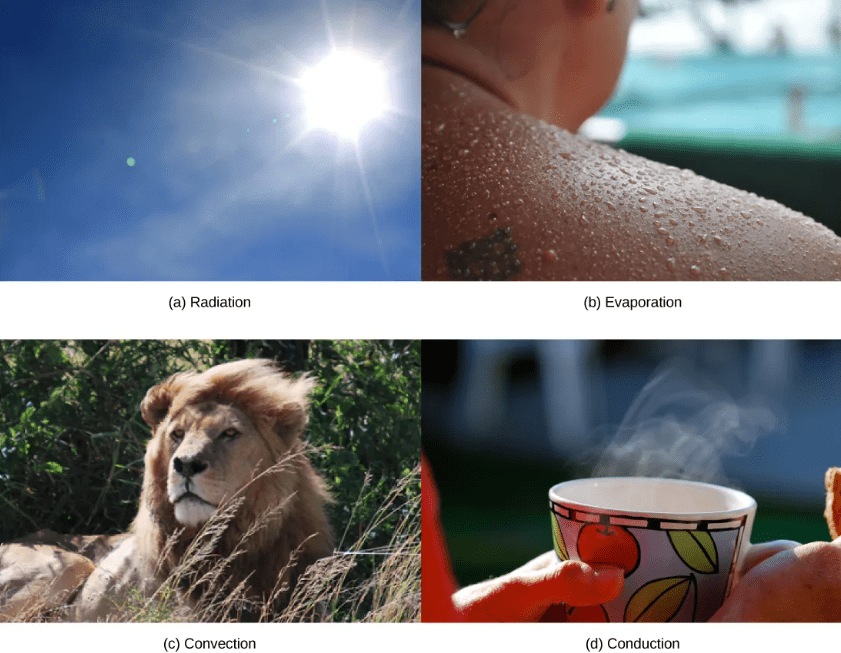
Animals take advantage of radiation, evaporation, convection, and conduction to conserve or dissipate heat in a variety of ways:
- Insulation, such as feathers, fur, or blubber, reduces the flow of heat between an animal and its environment. Many endothermic animals have some form of insulation. Animals with thick fur or feathers create an insulating layer of air between their skin and internal organs. Polar bears and seals live and swim in a subfreezing environment and yet maintain a constant, warm, body temperature. The arctic fox, for example, uses its fluffy tail as extra insulation when it curls up to sleep in cold weather. Mammals have a residual effect from shivering and increased muscle activity: arrector pili muscles cause “goose bumps” causing small hairs to stand up when the individual is cold; this has the intended effect of increasing body temperature. Mammals use layers of fat to achieve the same end. Loss of significant amounts of body fat will compromise an individual’s ability to conserve heat.
- Circulatory adaptations such as local vasodilation or vasoconstriction can help maintain body temperature in endotherms.
- Vasodilation brings more blood and heat to the body surface, facilitating radiation and evaporative heat loss, which helps to cool the body. It is estimated that African elephants can meet nearly 100% of their heat loss needs through vasodilation in the ears.
- Vasoconstriction reduces blood flow in peripheral blood vessels, forcing blood toward the core and the vital organs found there, and conserving heat.
- Some animals have adaptations to their circulatory system that enable them to transfer heat from arteries to veins, warming blood returning to the heart. This is called a countercurrent heat exchanger; it prevents the cold venous blood from cooling the heart and other internal organs. This adaptation can be shut down in some animals to prevent overheating the internal organs. The countercurrent adaptation is found in many animals, including dolphins, sharks, bony fish, bees, and hummingbirds.
- Metabolic waste heat is generated as a heat source by many animals, especially mammals. When muscles are contracted, most of the energy from the ATP used in muscle actions is wasted energy that translates into heat. Severe cold elicits a shivering reflex that generates heat for the body. Many species also have a type of adipose tissue called brown fat that specializes in generating heat. Many flying insects also “shiver” the flight muscles prior to flight in order to generate waste heat to warm the muscles.
- Perspiration or sweating is a form of evaporative heat loss common among mammals.
- Behavioral adaptations are common among both endothermic and ectothermic animals to help regulate body changes. For example, an animal in a hot desert environment may burrow underground during the hottest part of the day to keep from getting too warm. The same animals may climb onto rocks to capture heat during a cold desert night. Some animals seek water to aid evaporation in cooling them, including many reptiles, mammals, and birds. Animals can also engage in group behaviors such as the activity of bees to warm a hive to survive winter, or the huddling of emperor penguins against the wind to reduce convective heat loss.
Plant Responses and Adaptations to Water Stress
Plants require water for photosynthesis, and water stress in terms of either extreme flooding or extreme drought pose physiological challenges to plant survival. Flooded soil can cause plant roots to suffocate due to lack of oxygen to support cellular respiration. Plant vascular systems do not transport gases, so plant tissues typically must be in close proximity to air in order for the cells to exchange oxygen and carbon dioxide with the environment.
Too much water: Adaptations to growth in water-logged soil. Plants that are adapted to marshes and other aquatic areas often have one of several types of adaptations to facilitate gas exchange with the water-bound roots:
- Pneumatophore roots: Mangroves and other plants adapted to waterlogged soils have specialized root structures called pneumatophores that that extends from the underwater roots up above the water’s surface; the surface of each pneumatophore is covered with small pores that allow for gas exchange and transport of oxygen to the root tissue below water.

- Aerenchyma: Water lilies and other plants adapted to wetlands have modified parenchymal tissue called aerenchyma which create air channels in the leaves, shoots, and roots to facilitate transport of air from the stomata down to the roots under the water’s surface.
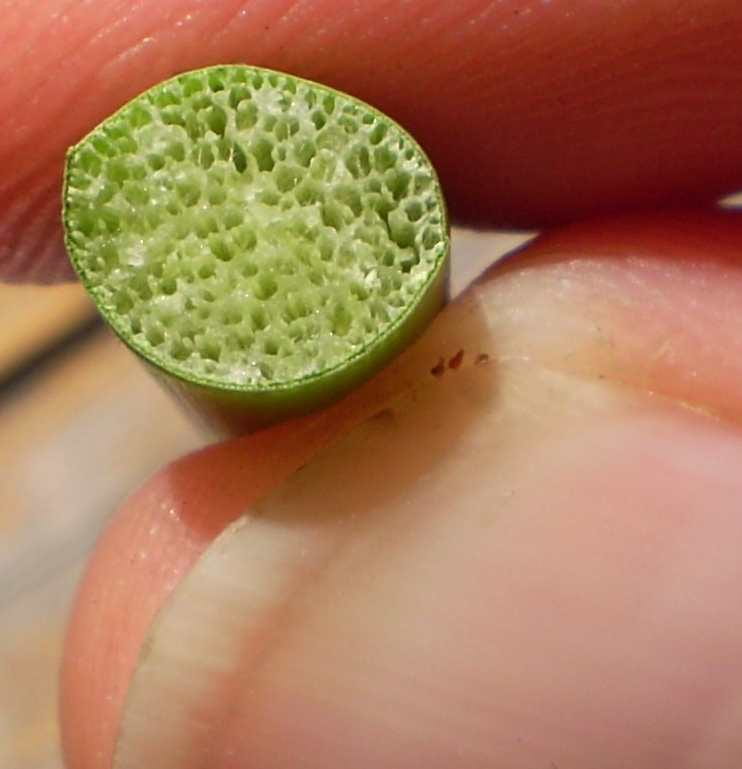
Too little water: Drought responses. Drought is also particularly challenging for a plant because the plant requires water to carry out photosynthesis, but photosynthesis results in water lost due to evaporation. Plants have different characteristic responses to short and long term drought (see Plant Hormones and Sensory Systems for a more detailed review):
- Responses to short term drought: Plants are covered in a waxy cuticle that prevents dehydration, and they exchange oxygen and carbon dioxide through openings called stomata, which are pores in the leaf surrounded by a pair of cells called guard cells. The hormone abscisic acid regulates the behavior of guard cells to open the stomata to allow gas exchange, or to close the stomata in response to low soil moisture levels associated with drought to prevent water loss and dehydration. Note that stomatal closure also prevents exchange of oxygen and carbon dioxide, which is necessary for both photosynthesis and cellular respiration.

- Responses to long term drought: Plants cannot keep stomata closed long-term, as they are unable to either photosynthesize or respire: plants will die if the stomata remain closed for too long. Thus if a drought persists for too long, the plant will begin sacrificing certain areas by allowing the leaves or stems to die in localized regions. This process may be regulated by the hormone ethylene, which can induce localized cell death resulting in dropping leaves or branches such that the plant is able to use its limited water resources to support a smaller body mass.
Adaptations to arid environments: Xerophytes are plants that are adapted to extremely dry, desert conditions, and include succulents like cacti and bromeliads like pineapples. Xerophytes may feature a wide array of adaptations to dry conditions such as thickened cuticles with epidermal hairs and sunken stomata which limit water loss, storage of water within their tissues, spines to dissipate heat, and leaf behaviors to curl or turn throughout the day to minimize full exposure to the sun. Alternatively, some xerophytes exhibit extremely short life cycles that they complete entirely within a brief rainy season.
C4 and CAM Plants: Photosynthetic Adaptations to Hot Conditions
The information below was adapted from Wikipedia “Photorespiration” and Wikipedia “Crassulacean acid metabolism”
Photosynthesis efficiency is dramatically reduced in hot and dry climates. Photosynthesis involves two separate reactions: the light reactions, which use light as the source of energy to collect electrons from water (producing oxygen as a byproduct) and produce ATP, and the Calvin-Benson cycle, which uses the electrons from the light reactions to reduce carbon dioxide.
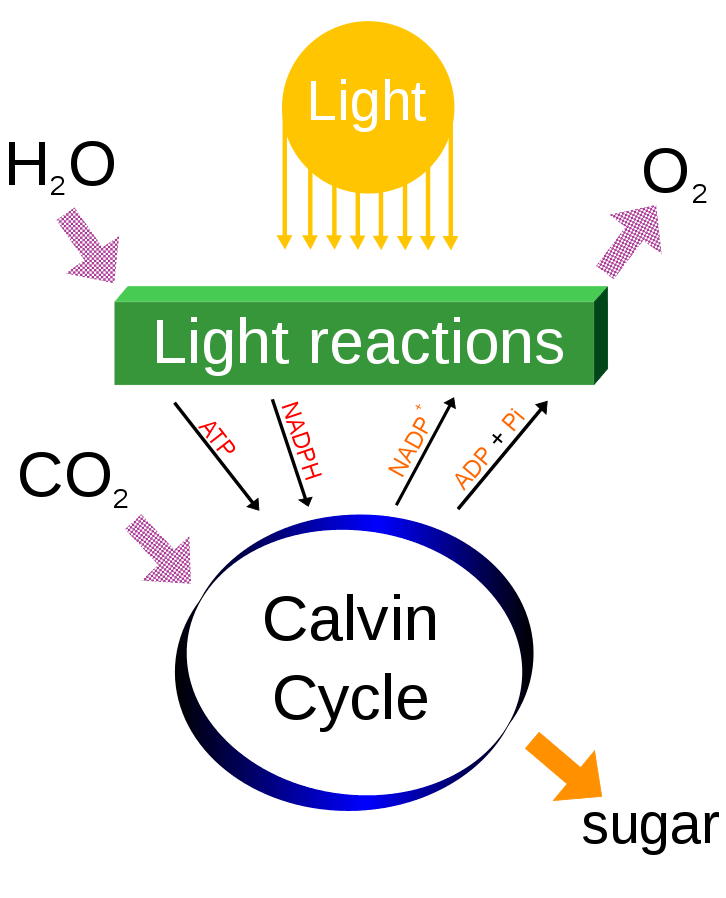
The enzyme rubisco, which is responsible for fixing carbon dioxide into glucose as part of the Calvin-Benson cycle, has poor substrate specificity and is unable to completely differentiate between molecular oxygen (O2) and carbon dioxide (CO2). When rubisco uses oxygen instead of carbon dioxide as a substrate, the result is process called photorespiration that wastes ATP generated by the light reactions and significantly reduces the efficiency of photosynthesis. The oxygenation reaction of rubisco is especially problematic for plants in hot and dry conditions for several reasons:
- The oxygenase activity of rubisco increases in hot conditions; that is, rubisco is worse at differentiating between oxygen and carbon dioxide when it is hot
- The reduced efficiency of photosynthesis means that plants require additional water for the light reactions to generate additional ATP to make up for what was lost due to photorespiration
- When stomata are closed during the heat of the day and/or during drought conditions, the concentration of carbon dioxide in the tissues decreases as it is used in the Calvin-Benson cycle, while oxygen continues to be produced by the light reactions; the increasing concentration of oxygen further increases the oxygenase activity of rubisco
The combination of these issues describe above are particularly problematic for plants in a hot climate experiencing drought, as they must use additional water to produce more energy from the light reactions even as they experienced reduced water availability due to drought. Many xerophytes (plants adapted to dry climates) use one of several mechanisms to “concentrate” carbon dioxide relative to oxygen within the plant tissue, to minimize the oxygenase activity of rubisco. These carbon concentrating mechanisms include two variations on photosynthesis, called the C4 pathway and the Crassulacean acid metabolism (CAM) pathway:
- C4 plants concentrate carbon dioxide by moving it deeper into the plant tissue into cells called bundle sheath cells, away from oxygen near the stomata. Unlike other plants, C4 plants only perform the Calvin-Benson cycle in the bundle sheath cells. C4 plants capture carbon dioxide entering the stomata using an enzyme called phosphoenolpyruvate carboxylase (PEP carboxylase) which catalyzes the combination of carbon dioxide with a compound called phosphoenolpyruvate (PEP), forming a 4-carbon sugar (hence the name “C4 pathway”) that is transported into the bundle sheath cells where oxygen concentration is low to avoid photorespiration. Here, carbon dioxide is freed from the 4-carbon sugar, and it enters the normal Calvin-Benson Cycle where it is reduced by rubisco to produce glucose. C4 plants include sugar cane, corn (maize), and sorghum.
- The take-home message is that C4 plants concentrate carbon dioxide by moving it (and the Calvin-Benson Cycle) deeper into the plant tissue where it is physically separated from the light reactions.
- CAM plants concentrate carbon dioxide by capturing carbon dioxide at night instead of during the day. CAM plants have their stomata closed during the day; at night, they open the stomata and use the enzyme PEP carboxylase to capture carbon dioxide and store it as a 4-carbon sugar, similar to the C4 pathway. The resulting organic acids are stored in vacuoles for later use, as the Calvin Benson cycle cannot operate without ATP and NADPH, products of light-dependent reactions that do not take place at night. During the day, the stomata close to conserve water, and the CO2-storing organic acids are released from the vacuoles. The CO2 is then freed from the organic acids and enters into the normal Calvin-Benson cycle where it is reduced by rubisco to produce glucose. CAM plants include pineapples, cacti, and other succulents.
- The take-home message is that CAM plants concentrate carbon dioxide by collecting it at night when the light reactions and the Calvin-Benson cycle cannot operate; the stomata close during the day to minimize water loss and the previously-collected carbon dioxide is then freed to enter the Calvin-Benson cycle.
The video below provides an overview of specific evolutionary adaptations in photosynthesis in C4 vs CAM plant species to that facilitate their survive in dry environments: