Learning Objectives
- Identify examples of and differentiate between sugar sources and sugar sinks in plant tissues
- Explain the roles of solute potential, pressure potential, and movement of water in the Pressure Flow Model for sugar translocation in phloem tissue
- Describe the roles of proton pumps, co-transporters, and facilitated diffusion in the Pressure Flow Model
- Recognize that the transport pathway used to load sugars at sources or unload sugars at sinks will depend on whether sugar is moving down or against its concentration gradient
- Compare and contrast the mechanisms and requirements of fluid transport in xylem and phloem
Sugars Move from “Sources” to “Sinks”
The information below was adapted from OpenStax Biology 30.5
Plants need an energy source to grow. In growing plants, sugars move from sites where they are produced (or stored) to sites where they are needed for growth (or storage) via a process called translocation, or movement of sugar through the plant phloem:
- A source is any structure in a plant that either produces (like a leaf) or releases (like a storage bulb) sugars for the growing plant.
- A sink is any location where sugar is delivered for use in a growing tissue or storage for later use. Growing tissues might include apical and lateral meristems or developing leaves, flowers, seeds, and fruits; storage locations might include roots, tubers, and bulbs.
Notice that a storage location might be either a source or a sink, depending on the plant’s stage of development and the season:
- In the middle of the growing season, the sources include mature leaves and stems which are actively photosynthesizing and producing excess sugars. Sinks during the growing season include areas of active growth meristems, new leaves, and reproductive structures like flowers and seeds. Sinks also include sugar storage locations, such as roots, tubers, or bulbs.
- At the end of the growing season, the plant will drop leaves and no longer have actively photosynthesizing tissues. Depending on the local climate, the growing season may end either due to onset of winter or onset of the dry season.
- Early at the start of the next growing season, a plant must resume growth after dormancy. Because the plant has no existing leaves, its only source of sugar for growth is the sugar stored in roots, tubers, or bulbs from the last growing season. These storage sites now serve as sources, while actively developing leaves are sinks. Once the leaves mature, they will become sources of sugar during the growing season.
The Pressure Flow Model for Sugar Translocation Requires the Movement of Water into and out of the Phloem
The information below was adapted from OpenStax Biology 30.5
The best-supported hypothesis to explain the movement of sugars in phloem is the pressure flow model for phloem transport. This hypothesis accounts for several observations:
- The fluid in phloem is under high positive pressure
- Translocation stops if the phloem tissue is killed
- Translocation can proceed in both directions simultaneously (but not within the same tube)
- Translocation is inhibited by compounds that stop ATP production in the sugar source
In very general terms, the pressure flow model works like this:
- A high concentration of sugar at the source creates a low solute potential (Ψs)
- The low solute potential draws water into the phloem from the adjacent xylem.
- Movement of water into the phloem creates a high pressure potential (Ψp), aka high turgor pressure, in the phloem.
- The high turgor pressure forces movement of phloem sap from source to sink through a process called “bulk flow.”
- The sugars moved via bulk flow are then rapidly removed from the phloem at the sink.
- Removal of the sugars at the sink then increases the Ψs, which causes water to leave the phloem and return to the xylem, which then decreases Ψp at the sink.
The video below provides a concise overview of sugar sources, sinks, and the pressure flow hypothesis:
Review of Transport Pathways in the Pressure Flow Model
Before we discuss more details of the pressure flow model, let’s first review some of the transport pathways we’ve previously discussed and add some new information to some of these transport pathways:
- Diffusion occurs when molecules move from an area of high concentration to an area of low concentration. Diffusion does not require energy because the molecules move down their concentration gradient (from areas of high to low concentration).
- Proton pumps use energy from ATP to create electrochemical gradients, with a high concentration of protons on one side of a plasma membrane. This electrochemical gradient can then be used as a source of energy to move other molecules against their concentration gradients via co-transporters.
- Co-transporters are channels that perform a type of secondary active (energy-requiring) transport. Co-transporters move two molecules at the same time: one molecule is transported along (“down”) its concentration gradient, which releases energy that is used to transport the other molecule against its concentration gradient.
- Symporters are a type of co-transporter that transports two molecules in the same direction: both into the cell, or both out of the cell.
- Antiporters are a type of co-transporter that transports two molecules in opposite directions: one into the cell, and the other out of the cell.
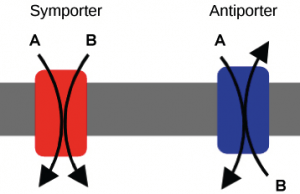
Transport Pathways in the Pressure Flow Model
The information below was adapted from OpenStax Biology 30.5
Photosynthates such as sucrose (a type of sugar) are produced in parenchyma cells of photosynthesizing leaves. Sugars are actively transported (requires ATP) from source cells into the sieve-tube companion cells, which are associated with the sieve-tube elements in the vascular bundles. This active transport of sugar into the companion cells allows the companion cells to accumulate a higher concentration of sugar than is present in the photosynthesizing leaves. To make this process work, the companion cells use an ATP-powered proton pump to create an electrochemical gradient outside of the cell; then a proton-sucrose cotransporter couples the movement of a proton down its concentration gradient with sucrose against its concentration gradient and into the companion cells.
Once inside the companion cells, the sugar diffuses down its concentration gradient from the companion cell and into the phloem sieve-tube elements through the plasmodesmata that link the companion cell to the sieve tube elements. Recall that phloem sieve-tube elements have reduced cytoplasmic contents and are connected by pores that allow for pressure-driven bulk flow, or translocation, of phloem sap.
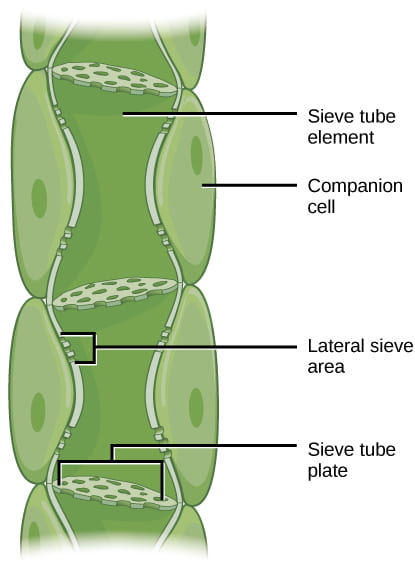
As noted in the previous section, the presence of high concentrations of sugar in the sieve tube elements drastically reduces Ψs; as a result, water then moves by osmosis from xylem into the phloem cells. This movement of water into the sieve tube cells then causes Ψp to increase, which increases both the turgor pressure in the phloem and the total water potential in the phloem at the source. This increase in water potential then drives the bulk flow of phloem from source to sink.
Unloading at the sink end of the phloem tube can occur either by diffusion, if the concentration of sucrose is lower at the sink than in the phloem, or by active transport, if the concentration of sucrose is higher at the sink than in the phloem:
- If the sink is an area of active growth, such as a new leaf or a reproductive structure, then the sucrose concentration in the sink cells is usually lower than in the phloem sieve-tube elements because the sink sucrose is rapidly metabolized for growth.
- If the sink is an area of storage where sugar is converted to starch, such as a root or bulb, then the sugar concentration in the sink is usually lower than in the phloem sieve-tube elements because the sink sucrose is rapidly converted to starch for storage.
- If the sink is an area of storage where the sugar is stored as sucrose, such as a sugar beet or sugar cane, then the sink may have a higher concentration of sugar than the phloem sieve-tube cells. In this situation, active transport by a proton-sucrose cotransporter (which relies on an ATP-powered proton pump) is typically used to transport sugar from the companion cells into storage vacuoles in the storage cells.
Once sugar is unloaded at the sink cells, the Ψs increases, causing water to diffuse by osmosis from the phloem back into the xylem. This movement of water out of the phloem causes Ψp to decrease, reducing the turgor pressure in the phloem at the sink and maintaining the direction of bulk flow from source to sink.
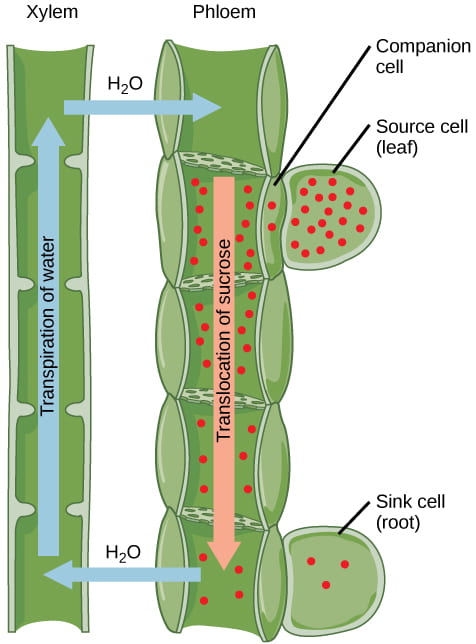
This video (beginning at 5:03) provides a more detailed discussion of the pressure flow hypothesis:
Movement of Fluid in Xylem vs Phloem
It should be clear that movement of sugars in phloem relies on the movement of water in phloem. But there are some important differences in the mechanisms of fluid movement in these two different vascular tissues:
- The “driving force” for fluid movement:
- Xylem: transpiration (evaporation) from leaves, combined with cohesion and tension of water in the vessel elements and tracheids (passive; no energy required)
- Phloem: Active transport of sucrose from source cells into phloem sieve tube elements (energy required)
- The cells facilitating fluid movement:
- Xylem: Non-living vessel elements and tracheids
- Phloem: Living sieve tube elements (supported by companion cells)
- Type of pressure potential
- Xylem: Negative due to pull from the top (Ψp decreases due to combined effects of transpiration combined with cohesion and tension of water)
- Phloem: Positive due to push from source (Ψp increases due to influx of water which increases turgor pressure at source)